Fasteners
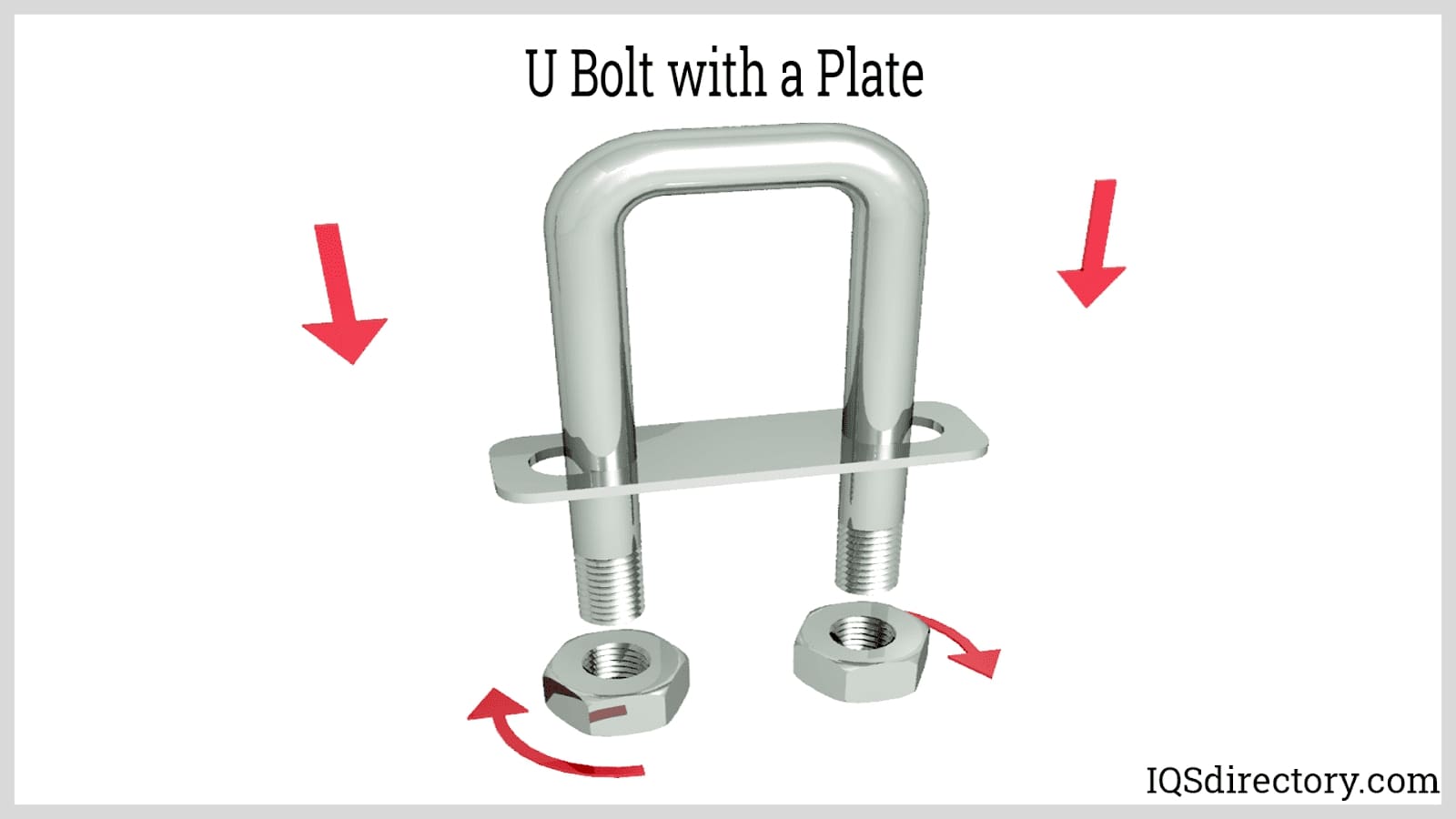
A fastener is a non-permanent or permanent mechanical tool that rigidly connects or affixes two surfaces or objects together. Non-permanent fasteners can easily be removed without damaging the joined materials...
Please fill out the following form to submit a Request for Quote to any of the following companies listed on
This article will take an in-depth look at cold heading and cold forming.
The article will bring more detail on topics such as:
This chapter will cover the definition of cold forming, the materials used, and the various tooling techniques involved in the process.
Cold heading, often referred to as cold forming, involves creating a specific shape or design from metal wire without applying heat. This process is facilitated by high-speed hammers, dies, and punches to shape the metal continuously.
Cold forming starts with a slug cut from a coil or wire, which is then shaped by a cold heading machine using a series of powerful hammers and dies. This process can also be described as applying force from a punch to the end of a metal blank within a die. To achieve plastic flow, the applied force must exceed the metal’s elastic limit or yield strength.
Cold heading can be considered a forging operation without the use of heat. It is performed together with other cold heading processes such as blank rolling, piercing, pointing, thread rolling, sizing, and trimming. Cold heading is also an industrial process that acts as the primary way to make metal threaded fittings and fasteners.
Cold heading is a high-speed forging process where coiled wire is precisely cut to length at room temperature and under the appropriate pressure. The wire is then fed through a series of continuous die and tool openings, which shape and displace the metal to form the desired product. This process allows for the adjustment of feedstock diameters and lengths and includes operations such as piercing and trimming to remove small material amounts. All cold heading procedures are performed in a streamlined, continuous operation.
Historically, cold heading was only used for making very simple fasteners. Currently, cold heading is an automated high-speed and multi-station method that is able to economically produce composite fasteners and other components. With the improvement of technology and growth in cold heading, the equipment has the ability to cold form parts from tougher metals, including higher-temperature alloys and stainless steels.
Cold headers utilize a specific technique to cut and measure segments from a wire coil. The method for feeding the wire into the cold header and cutting it off can vary depending on the machine's brand. Inside the machine, a cut-off knife is employed, which strikes the wire from a predetermined angle to sever a single piece. This process typically involves multiple components in addition to the cut-off knife.
Dies are significantly larger than punches due to the need to endure greater loads. They are positioned on the stationary section of the cold header and typically consist of several pieces. The working tool of a die is often assembled from three to four segments that collectively form the complete working component. These segments are then subjected to high pressure within a large, curved container, commonly referred to as the casing.
The die's inserted part endures intense radial pressure with each blow. Without adequate support, it would quickly fail or explode. To prevent this, the die is securely forced into a casing. This casing, which is much larger, absorbs and disperses the accumulating pressure, maintaining the die's integrity over many parts or cycles. Die casings can be reused multiple times before needing replacement.
Each die features a precisely sized central hole aligned with the tool's axis where the part is positioned. To prevent the part from being pushed out through the back of the die under high pressure, a knock-out pin system is employed. This system consists of a series of pins located at the rear of the die. During the initial phase of the stroke, these pins prevent the part from being expelled. In the final phase of the forming stroke, the pins are driven forward to eject the finished part from the die.
Punches are located on the moving part of the cold heading machine. They are generally smaller than dies because they deal with less pressure during the forming process. Despite their smaller size, punches still experience significant loading and are housed within punch casings for protection.
Punches are used to shape or form the head of screws. Typically, one punch is used for the initial impact, while another is used for the final impact. Additionally, punches can act as supports for pins that help push the part into the die. This is particularly useful when dealing with trapped extrusions or recess pins, which facilitate backward extrusion to create recesses in parts.
The transfer mechanism is the final tool system in cold forming. The process is straightforward in a single-die, two-blow machine, as only one transfer is required—moving the part to the next die. However, in multi-die headers, where the process involves several dies, the transfer mechanism becomes crucial.
Commonly used tooling components for transfer include fingers or grips that securely hold parts as they move between dies. These fingers are often custom-designed to suit the specific requirements of each part. Proper part geometry is critical for successful transfer, especially since parts can be quite heavy. Improper transfer can lead to issues such as head upset or shoulder droop as the part exits the die.
The operator must precisely time the transfer to ensure that the part is securely held before it fully exits the die. The transfer mechanism is designed to wait until the part is completely clear of the die before moving it. Mastery of this process requires significant practice and expertise from the setup technician.
Cold heading machines transform a metal blank into precisely shaped parts using a reciprocating ram that drives a hammer into a preformed die. In mechanical processing and manufacturing, these machines are commonly referred to as stamping machines. Provided that the initial raw materials remain unchanged, they are shaped according to the desired specifications.
In a cold forming machine, bevel gears are paired and put on one end of the crankshaft. The driven bevel gear shaft is put on a cam gear, with a scissors slider vertically put on the roller shaft consisting of the driven roller of the cam object or device. The utility model overcomes all the encountered deficiencies of the prior art, and also the moving friction coefficient. The impact force among all components of the cutting and feeding mechanism are very small in magnitude. The smooth operation of the equipment largely minimizes the operating noise and improves product productivity and quality.
Cold heading employs high-speed automated equipment known as cold headers or part formers. This machinery can convert a basic wire into complexly designed components with precise and consistent tolerances at rates up to 400 pieces per minute. A measured length of wire is placed into a die, where a hammer then shapes or deforms the material to achieve the desired form.
Cold heading is a volume-specific process where the material's volume remains constant throughout the shaping process. The metal is merely rearranged to form a precisely designed part with the same volume, resulting in minimal material loss or scrap.
Recent advancements in cold heading and cold forming machinery have significantly enhanced their capabilities. Modern machines now feature five or more dies and include various functionalities to optimize the production of both short and long parts. Operators can make single adjustments to modify the feed stroke, cutter, kickout, and transfer timing functions. With CNC controls, production data is readily accessible, enabling quick setup changes. This allows for efficient adjustment of both die and punch components, minimizing downtime and maximizing production efficiency. Additionally, multi-station headers are increasingly utilized for their versatility.
Modern cold forming operations integrate a range of techniques including extruding, upsetting, and other processes to enhance production efficiency. Advances in metallurgical science have significantly improved the ability to cold form tougher and harder materials. Leading alloy manufacturers meticulously control the composition and production of alloys to meet the growing demand for higher strength and improved corrosion resistance in cold-formed components.
Specific AISI grades can be engineered within controlled parameters to enhance cold formability, ensuring superior performance and ease of subsequent machining. The refined analysis of cold forming processes also allows for the economical production of specialized fastener shapes. Additionally, the development of more durable tool steels has extended the lifespan of heading dies, while versatile alloys are designed to be suitable for both machining and cold heading applications.
Cold heading equipment generally falls into two primary categories:
Both types of cold heading equipment utilize horizontal reciprocating rams and stationary bolsters. Cold heading machines can range from single-die setups to those with up to seven dies in sequence, accommodating various cut-off sizes. The capability of a manufacturer in cold heading largely depends on the number and types of machines they have in operation.
The cold heading tooling process primarily involves two key components: dies and punches. Punches apply the necessary force from the machine to the material, ensuring precise dimensional control of the part features. Meanwhile, dies guide and shape the material into the desired form and dimensions.
The term "cold heading" originates from the upsetting process used in the technique.
Cold heading is the technique employed to form a head on a bolt or screw. Upsetting refers to the process of consolidating material into a specific area of the component, like a collar or head. In essence, this process enlarges the diameter in the areas being worked. In cold heading terminology, manufacturers often refer to this as "the maximum number of diameters that can be upset."
Although it looks and sounds much more complex, upsetting is a simpler way of forming. It is a simple cylinder that is cut or removed from the lengthy continuous wire coil that is put inside the machine. For the parts that will become bolts and screws, or the externally threaded ones, the cylinder is much longer than its diameter. It is possible to break the cylinder down into different sections where each section is the same diameter and height. Each division is called a diameter. Some parts are very long and skinny, such as screws and bolts, with each beginning piece of wire consisting of multiple diameter sections.
Engineers have discovered that only a limited amount of material can be manipulated or compressed in one go before it risks bursting or cracking. In cold heading processes, this limit is generally about two percent of the diameter per strike. Given that many bolt heads or screws contain more than two diameters' worth of material, multiple strikes are necessary to achieve the desired result.
There is a constraint on how much material can be upset in a single blow under controlled conditions. For forming complex parts where more metal needs to be moved, the process is often conducted in two stages or blows. This explains the prevalence of single-die and double-stroke headers. Upsets are typically calculated based on wire diameters, dividing the total length of the metal blank by the wire diameter.
For instance, a 5-inch metal blank made from 1/2-inch wire corresponds to ten diameters in length. On a solid die header, the maximum dimension of wire that can be upset in a single blow is approximately 2 1/4 diameters. Thus, around 1 inch of the 5-inch metal blank can be used to form the required fastener head. Generally, single blow heading falls within the range of 1 to 1 1/4 diameters.
In a two-blow heading process, up to 4 1/2 diameters can be upset. During the initial contact between the metal blank and the punch, any part of the blank extending unsupported out of the die may bend if struck, leading to a cold shut defect. Attempting to upset a length of 3 inches, equivalent to six diameters, may be unmanageable.
However, there are exceptions to this rule. Advanced headers with sliding punches can accommodate up to 6 1/2 inches in diameter for two-blow upsets. In multi-station headers, the total diameters that can be upset are only limited by the number of available dies. Properly matching the wire diameter to the machine’s capabilities is crucial, as incorrect calculations can lead to mismatches and ineffective forming.
In the context of cold heading, "extrusion" refers to several distinct processes. There are primarily three types of extrusion: forward extrusion (which includes trapped and open extrusion), and reverse extrusion. In forward extrusion, the material is pushed forward through the die, either moving out in front of the tool (trapped extrusion) or extending outward in an open manner. Reverse extrusion, on the other hand, involves the material being forced backward, against the tool’s direction.
For instance, in forward extrusion, a metal blank is pushed into a die with a significantly smaller diameter than the blank itself, leading to an increase in length and a reduction in diameter. Conversely, in backward extrusion, the metal blank is subjected to high pressure from a punch at an angle. With no other path available, the material is forced along the edge of the punch and moves backward.
Forward extrusion is typically employed for producing items such as screws, stepped shafts, or bolts, while backward extrusion is commonly used for creating various cylindrical shapes like sleeves, nuts, and rivets. Similar to upsetting, extrusion alters the shape of the metal blank without wasting material. The extrusion process can be classified as either open or contained.
In open extrusion, the blank is driven into a die, whereas in trapped extrusion, the blank is entirely enclosed within the die. The effectiveness of controlled upsetting processes is influenced by the wire diameters, while extrusion processes depend on the reduction of the blank's area, often expressed as a percentage and determined by the extrusion angle.
A general guideline for open extrusion is that the area reduction in a single blow should not exceed 30 percent. It’s important to remember that the diameter measurements before and after extrusion are not used for calculating the area reduction directly.
In open extrusion, the part isn't confined by tools, allowing the extruding section of the tool to be positioned near the top of the die. This setup maximizes the length of material that can be extruded from the part.
However, open extrusion has its drawbacks. It requires substantial pressure to force the material through the extruding tool. Without adequate support from the tools, excessive pressure can lead to buckling, which can cause the process to fail.
Trapped extrusions, in contrast to open extrusions, involve completely enclosing the part within the tool before it is pushed through the extruding section. This method allows for a significant reduction in diameter, up to about seventy-five percent, due to the increased pressure that can be applied.
However, a limitation of trapped extrusion is that it requires a substantial portion of the tool to be occupied to create sufficient space for the extrusion. As a result, the length of the extruded part tends to be shorter and more restricted compared to open extrusion. Trapped extrusion, also known as contained extrusion, is crucial for producing complex parts in headers.
The radial extrusion die is often preferred for contained extrusion of the cold headed parts. This is because this form of die decreases die pressure and improves the pattern of flow of the wire as it is driven through the die. With almost all contained extrusion dies, the best results are obtained by making wire that has a larger diameter than the shank that is finished.
The wire gauge is chosen with precision to ensure that the resulting head dimensions are approximately twice the diameter of the original wire. The wire is then extruded to match the diameter of the shank and upset to achieve the specified head dimensions using standard techniques.
Reverse extrusion is primarily used to create hollow components like bushings and nuts. In this process, a pin is pushed into the material inside the die. There is considerable clearance between the pin's outer diameter and the die walls, allowing the material to flow backward along the pin. This results in the formation of an outer shape and the beginning of an internal channel or hole.
Reverse extrusion is also employed to create internal drive recesses in various screw heads. The final stages in cold heading include trimming and piercing, though not all parts undergo these processes. In fact, many externally threaded screws do not require trimming.
Both trimming and piercing involve shearing techniques where tools apply force, design, and speed to remove material from the targeted area. Trimming is generally reserved for finishing touches on collars or head shapes, such as creating a hex head. For internally threaded components, the reverse extrusion process is typically used on all sides, resulting in a blank with holes that do not extend fully through the part, separated by a thin web of material.
The piercing process follows, where a pin is driven into the hole, completely shearing the web to create a continuous hole through the part. In both cases, whether trimming an external part or piercing an internal part, the resulting scrap is minimal. This offal represents only a tiny fraction of the total material used in cold heading.
This chapter will discuss the applications and benefits of cold heading forming.
The cold heading process manufactures fasteners with high efficiency and good quality, saving costs and materials. However, this process requires high quality raw materials.
Cold heading utilizes a specific grade of steel known as cold heading steel, ideal for producing fasteners and joints. Additionally, this process can be applied to tempered alloy steels, structural steels, and ferritic-martensitic duplex steels.
Cold forming offers several key benefits, including:
Cold forming is known for its rapid production capabilities, with some manufacturers achieving output rates of up to 100 parts per minute. This high-speed process ensures uniform quality and accuracy in the finished parts.
Cold forming enhances the strength of the part by maintaining the material's inherent properties. Since the material is shaped without cutting or heating, its original grain structure aligns with the part’s contours, resulting in increased durability and strength.
Unlike hot forging, the cold forming process does not require extra energy to heat the material, making it more energy-efficient.
There are several limitations associated with cold heading, including:
As the diameter of a fastener increases, the pressure needed to shape the material also rises. While large machines are capable of producing fasteners of any size, they are often not practical for such tasks. For extremely large fasteners, machining is typically the more suitable method.
Not all materials are equally suited for cold forming. While various metals can be used, selecting the most appropriate types will yield superior results and enhance the quality of the final products.
Over the years, the process of cold heading has greatly helped with improving technology and producing higher-quality machining results. Thus, when selecting cold forming for a particular process, it is vital to understand its applications, benefits, and how each cold heading approach functions.
A fastener is a non-permanent or permanent mechanical tool that rigidly connects or affixes two surfaces or objects together. Non-permanent fasteners can easily be removed without damaging the joined materials...
Powder metallurgy is a manufacturing process that produces precision and highly accurate parts by pressing powdered metals and alloys into a rigid die under extreme pressure. With the development and implementation of technological advances...
Screw machined parts are complex pieces that are typically cylindrical and threaded and a screw machined product is a family of automatic lathes for small to medium-sized components...
A self-drilling screw is a type of tool that has the same design features as a drill bit or cutting tool. As the name implies, self-drilling screws do not require a pilot hole in order to perform as a fastener...
Swiss screw machining is a process for producing high precision parts in high volume using an automatic lathe that is programmed to perform every aspect of the cutting process. The method used for...
Thread rolling is a type of threading process which involves deforming a metal stock by rolling it through dies. This process forms external threads along the surface of the metal stock...
Bolts are tools used to fasten and secure the faces of assemblies using a nut. They are threaded and come with a matching nut. Their purpose is to connect aligned unthreaded holes by being placed through...