Strain Gauges
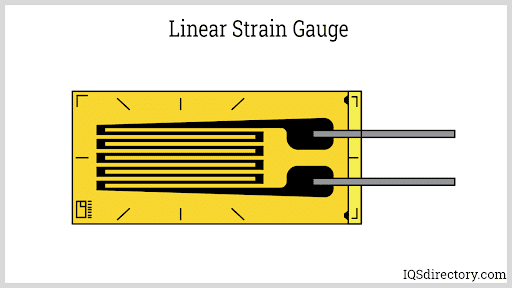
A strain gauge, or strain gage, is a sensing device used for measuring strain experienced by an object. It is made from a long, thin piece of conductor bonded to an elastic backing material called a carrier...
Please fill out the following form to submit a Request for Quote to any of the following companies listed on
This article will take an in-depth look at force sensors.
The article will bring more detail on topics such as:
In this chapter, we will delve into the mechanics of force sensors, discussing their functionality and essential considerations when selecting them for your needs.
Force sensors are instruments that transform various mechanical forces—such as weight, tension, compression, torque, strain, stress, or pressure—into electrical signals. These signals quantify the force's magnitude and can inform operators or function as inputs for machinery and process controls. They often transmit data to indicators, controllers, or computer systems.
While technically different from force transducers, the terms are usually used interchangeably. Force sensors are increasingly critical in power equipment, engineering machinery, various operating machines, and industrial automation. They vary in size, capable of measuring forces from mere grams to several tons.
Applications for force sensors cover a diverse range, from bathroom scales and musical instruments to medical devices, automotive seat occupancy detection, and industry-specific process controls. We'll discuss different force sensor types and elucidate their working principles.
Force sensors function by detecting an applied force and converting it into a quantifiable measurement. Various force sensor types exist, each with unique sensing mechanisms. For instance, some materials like force-sensing resistors alter their resistance when force is applied.
Typically, force sensors comprise electrodes and a sensing material. They determine the applied force by sensing changes in the resistance values of force-sensing resistors, the cornerstone of most force sensor designs.
Force-sensing resistors utilize contact resistance principles. In these resistors, a conductive polymer sheet changes its resistance when force is exerted. This sheet contains a matrix of tiny, electrically conductive, and non-conductive particles.
Applying force to the film's surface causes microscopic particles to make contact with the sensor electrodes, altering the film's resistance. This resistance change indicates the applied force's magnitude, where the mechanical pressure modifies the electrical resistance, providing measurable data.
Force sensors vary in design and applicability. Chapter three will detail the different types of force sensors. Meanwhile, in this section, we highlight critical factors to consider when selecting a sensor, aiding in informed decisions after evaluating your options.
The cost is a vital consideration when choosing a sensor. Depending on the application, expensive sensors could affect the project's financial viability.
It's important to consider if the variable being measured can repeatedly produce the same results under consistent conditions. Repeatability gauges a sensor’s capability to consistently deliver identical results, even if not perfectly accurate, under unchanged settings.
Accuracy and precision are significant specifications. Although sometimes interchanged in sensor advertising, they denote different things. Grasping the variance between them is crucial for deciding which is more pertinent to your needs.
Choose a force sensor based on its operational environment. Factors like temperature and humidity can influence sensor performance in industrial, agricultural, medical, or automotive contexts, potentially affecting accuracy.
Temperature fluctuations can drastically affect sensor outputs, though some sensors are built to withstand such variations. Choosing a resilient sensor is key to dependable performance in its destined environment.
A sensor's durability is crucial. Assess its robustness, lifespan, and resilience to harsh conditions. Every sensor has a limited life span, often defined by its performance over time or during storage.
Factors such as design, materials, manufacturing process, and environmental influences affect sensor longevity. Ensuring sensors are durable helps maintain cost-efficiency and suitability, reducing accuracy drift under disadvantageous conditions.
Choosing a force sensor with an appropriate response time is critical. Rapidly shifting forces require a sensor with a quick response time, whereas steady or slow forces might be sufficiently measured with slower response times.
Comprehending your project's specific needs is vital when selecting a force sensor. Open load sensors are some of the most precise available and can be customized for diverse applications.
The force-sensing technology selection will highly depend on the size and shape the product requires.
This chapter will cover the construction, design, and manufacturing processes of force sensors.
Depending on the intended usage, force sensors can have a wide range of sizes and shapes. A force sensor consists of the following three fundamental parts. Last but not least, the circuit section is typically formed of enameled wire or PCB board, with the force-sensitive components typically composed of aluminum alloy, alloy steel, and stainless steel. The circuit is created by connecting these gauges across the sensing element; the conversion element is frequently a strain gauge made of a coil. To achieve the same outcomes, it is also possible to use a piezoelectric sensor that runs on a crystal.
Force sensors can output in various formats, including analog voltage, analog current, analog frequency, switches or alarms, and serial or parallel data. In basic designs, the measuring circuit typically comprises four gauges. More complex sensors can include up to thirty gauges in their measurement circuits. The sensitivity of a force sensor to detect and track measurement deviations improves with the number of gauges. These gauges and circuits are generally designed based on the Wheatstone bridge equation.
The Wheatstone bridge, with its diamond-shaped circuit and variable resistance, is used for accurately measuring unknown resistance values or calibrating measuring devices like sensors. It allows for the measurement of very low resistance values, down to the milli-Ohm range, by comparing an unknown resistance against known resistances to determine its value.
With modern operational amplifiers, the Wheatstone Bridge Circuit can be integrated with various transducers and sensors, expanding its range of applications. The circuit features two series-parallel resistance configurations connected between a voltage supply and ground. When balanced, it produces no voltage difference between the two parallel branches. The circuit includes two input terminals, two output terminals, and four resistors arranged in a diamond pattern.
Initially, silicon nitride is applied to both sides of an insulator wafer. The front-side nitride is then etched away, exposing most of the thin silicon on the front side while leaving a nitride ring around the edge, using a photolithographically patterned etch mask and a reactive-ion etcher.
Next, silicon oxide is deposited on the front side to protect the semiconducting silicon beneath it. The nitride on the back side is then etched to form square windows that resemble diaphragms. Silicon is anisotropically etched with potassium hydroxide (KOH), using the nitride as an etch mask and the original oxide as an etch stop. After the KOH etching, the square silicon diaphragms are suspended over open cavities, which are subsequently sealed with Pyrex wafers.
Channels are etched into the Pyrex wafer using hydrofluoric acid and a chromium-gold etch mask. The Pyrex wafer features a clean, flat back and an engraved front. Once the SOI wafer and Pyrex wafer are anodically bonded, resistors are etched onto a metal layer that has been deposited on the front side through thermal evaporation.
To ensure waterproofing and electrical insulation of the resistors from the surrounding environment, a thin layer of Parylene polymer is applied to the front side. Individual pressure-sensing cells or complete pressure-sensing arrays are then separated from the bonded wafer pair using a die saw before packaging for testing. The fabrication sequence is critical: metal patterning must occur after its initial deposition onto the wafer but before KOH-etching and bonding. Metal patterning cannot be done between KOH-etching and bonding.
To detect and measure the minute resistance changes, a Wheatstone bridge circuit is used, as the resistance variations are very small. This setup is employed in both piezoresistive and strain-gauge pressure sensors.
Generally, there are four main types of force sensor technologies, classified by the behavior of their sensing elements. These methods—capacitive, inductive, piezoelectric, and piezoresistive—are effective in a range of environmental conditions.
An inductive sensor operates on the principle of electromagnetic induction to detect or measure objects. When the magnetic field around an inductor changes, a current flows through the circuit containing the inductor, in contrast to the case where a current through an inductor generates a magnetic field.
This effect allows the detection of metal objects interacting with a magnetic field. Inductive sensors are suitable for use in damp or dirty environments because non-metallic substances, like liquids or certain types of dirt, do not affect the magnetic field. Some inductive sensors adjust their characteristics to measure forces, such as through self-inductance, mutual inductance, or the generation of Eddy currents.
Inductive force sensors are particularly effective for low force readings and displacement measurements. The development of bi-directional inductive force sensors allows for the measurement of both the amplitude and direction of force in a plane. Eddy currents are induced on the surface of a conductor by the AC magnetic field of an LC sensor, which counteracts the magnetic field, thus reducing the sensor's inductance. This reduction in inductance varies with distance.
The oscillator generates a high-frequency electromagnetic field that is emitted from the sensing face of the switch. When conductive metal interacts with this field, it induces Eddy currents within the metal, altering the amplitude of the oscillations. This change results in a voltage variation at the output of the oscillator, which in turn affects the trigger's state and the output status.
Magnetic sensors are activated by the presence of a permanent magnet. They operate based on reed contacts, which consist of thin plates sealed in a glass bulb filled with inert gas. When a magnetic field is applied, these plates bend and make contact with each other, establishing an electrical connection. The surfaces of these plates are coated with a material optimized for low current or high inductive circuits.
Magnetic sensors offer several advantages over traditional mechanical switches: The hermetic glass bulb and inert gas provide excellent protection against dust, oxidation, and corrosion; the contacts are actuated by a magnetic field rather than mechanical movement. Additionally, the special surface treatment of the contacts ensures a long service life.
Capacitive sensing involves measuring electrical capacitance between conductors in a dielectric medium. This technique can detect material properties such as the dielectric constant. It is well-suited for integrated circuits, enabling the creation of compact sensors. Capacitive sensors are used in various applications, including measuring pressure, distance, thickness, proximity, and touch.
Analog capacitive sensors operate similarly to standard capacitive sensors but provide distinct advantages depending on their application. Compared to traditional capacitive proximity sensors, analog capacitive sensors offer benefits such as versatility in different environments and effectiveness in monitoring material selection, product thickness, concentration changes, and proximity detection.
This type of capacitive sensor is designed to fit into very tight spaces due to its compact shape. It operates similarly to detectors used for counting tasks and is mainly employed to monitor and control machine processes. To ensure high precision in confined areas, these small sensor heads need an additional amplifier. The external amplifier's potentiometer allows for adjusting the sensitivity as needed.
High-temperature capacitive sensors are used in environments where the sensor head is exposed to a broad temperature range. These sensors can accurately detect the temperatures of liquids and bulk materials, even in direct contact with very hot substances and extreme conditions.
A piezoelectric force sensor is a device that leverages the piezoelectric effect. Certain materials generate an electrical voltage when subjected to mechanical stress or forces applied along particular axes.
Resistive force sensors change their resistance in response to various environmental factors such as force, strain, and pressure. These sensors use a strain gauge, which can be either integrated into the sensor or attached to it. When an external force is applied, the resistive element deforms relative to the substrate. The conductive material on the substrate contacts the active area when air from the spacer aperture is forced through the air vent in the tail, leading to improved conduction and a decrease in the material's resistance. This variation in resistance allows the sensor to monitor and provide output based on the applied force, strain, and pressure.
Flexiforce touch force sensors are easy to incorporate into force measurement applications, where they measure the force applied between two surfaces. They can also determine the extent of bending using flex sensors. According to the bending strip theory, these sensors detect changes in resistance when the strip is bent. They can be interfaced with any controller for measurement. As the sensor bends, its resistance changes, functioning similarly to a variable resistor. Flexiforce sensors are characterized by their thin, flexible printed circuits.
A strain gauge sensor uses elastic deformation of the strain gauge to convert an applied force to an electrical signal. Both static and dynamic forces may be present. Weight, acceleration, and pressure are a few examples. The strain gauge material's internal deformation alters the gauge's internal dimensions.
When force is applied parallel to the thin wiring of the gauge and is tensile, the wire elongates and becomes narrower, similar to stretching a rubber band. This results in an increase in the gauge's resistance. Conversely, compressive forces cause the wire to widen and shorten, leading to a decrease in resistance. The change in the material's electrical resistance is directly proportional to the amount of applied force in both stretching and compressing directions.
Optical pressure sensors detect pressure changes by observing their effect on light. In its simplest form, a mechanical device may dim the light as pressure increases. More advanced sensors achieve high accuracy in measuring minute pressure changes through phase difference measurements.
In an intensity-based optical pressure sensor, increased pressure progressively obstructs the light source. This obstruction causes a reduction in the amount of light received, as the pressure moves a diaphragm and an opaque vane dims the LED’s brightness. The decrease in light intensity detected by the photodiode directly correlates with the applied pressure.
For basic optical pressure sensors, a reference photodiode that remains unobstructed by the vane is essential. This allows the sensor to adjust for variations in supply voltage and changes in light output due to external factors.
This chapter will explore the applications of force sensors, along with their benefits and limitations.
In industrial settings, force measurement is crucial, with force transducers frequently used to gauge forces for weight measurement or during production processes. These transducers are essential for metering forces in industrial machinery and systems, including presses, assembly lines, and end-of-line inspections.
Scales are among the most typical applications for force sensors. Scales come in a wide variety of designs, including counting scales, bench scales, hopper scales, platform scales, truck scales, belt scales, and more. In order to provide accurate results when weighing different materials, force sensors are used.
Onboard weighing involves measuring the load of heavy goods or industrial vehicles while they are still loaded. This method ensures that vehicles operate at their optimal capacity—neither under-loaded, which could lead to inefficiency, nor over-loaded, which could be hazardous.
In grocery stores, scales integrated into self-service checkout counters use force sensors to weigh items during the scanning process.
Luggage scales at airports are another common application of force sensors. In the medical field, force sensors are vital for various functions, including monitoring fluid levels, operating MRI and dialysis machines, performing endoscopic procedures, and supporting physical therapy equipment. These sensors used in medical contexts must comply with specific regulatory standards. In the automotive industry, force sensors are crucial for accurately measuring truck axle loads, which ensures effective and timely load management.
Automobiles utilize numerous force sensors. For example, sensors located near trailer couplings can provide important data regarding static weight relative to dynamic driving conditions. This capability allows vehicle control systems to adjust more efficiently and effectively. In the railway sector, force sensors are used in several applications.
Force sensors measure cargo and passenger weights, as well as tractive forces on drawbars. This data is crucial for ensuring safe and efficient operations within the prescribed limits. Additionally, force sensors are employed in railway track systems.
In aerospace, force sensors are used for various purposes, including providing feedback, detecting flap positions, supporting autopilot functions, and recording flight data.
Force sensors assess the forces exerted on objects, such as in testing the impact of an accident on a motorcycle helmet.
Reference measurements are used to ensure consistency in measured values across different regions. Metrological institutes worldwide test systems using high-precision force transducers and provide standardized reference values for both domestic and international contexts.
Test benches frequently utilize force transducers to verify that materials receive the appropriate load during testing.
This section will outline the pros and cons of the various force sensor types discussed in Chapter Three. Understanding these can assist in selecting the most suitable force sensor for specific applications by providing a comparative overview.
Due to the need for force measurements in numerous sectors, including instrumentation, robotics, medicine, artificial intelligence, vehicles, and wireless transmission, to name a few, force sensing technology is of great interest to researchers. Additionally, force sensing is a difficult task since the measured force has been in a range of different scales and is affected by outside factors including noise, the environment, and material qualities. This study effectively presented a variety of force sensing technologies and its optimization approaches, and also assessed a variety of force sensor applications in diverse fields.
A strain gauge, or strain gage, is a sensing device used for measuring strain experienced by an object. It is made from a long, thin piece of conductor bonded to an elastic backing material called a carrier...
A load pin is a sensor utilized to measure force or weight in various research, control, measurement and testing applications. The load pin force sensor converts a force into an electrical signal. The load pins provide durable and...
At the heart of every weighing device is a sensor called a load cell. When an item is put on a load cell, it senses the force of the gravitational pull of the weight, which an electronic circuit processes to display as data...
A platform scale is a scale that measures the weight of objects loaded on a flat platform. The function of the platform is to transmit the weight of the object to the internal measuring device and to support the object during weighing...