Pressure Vessels
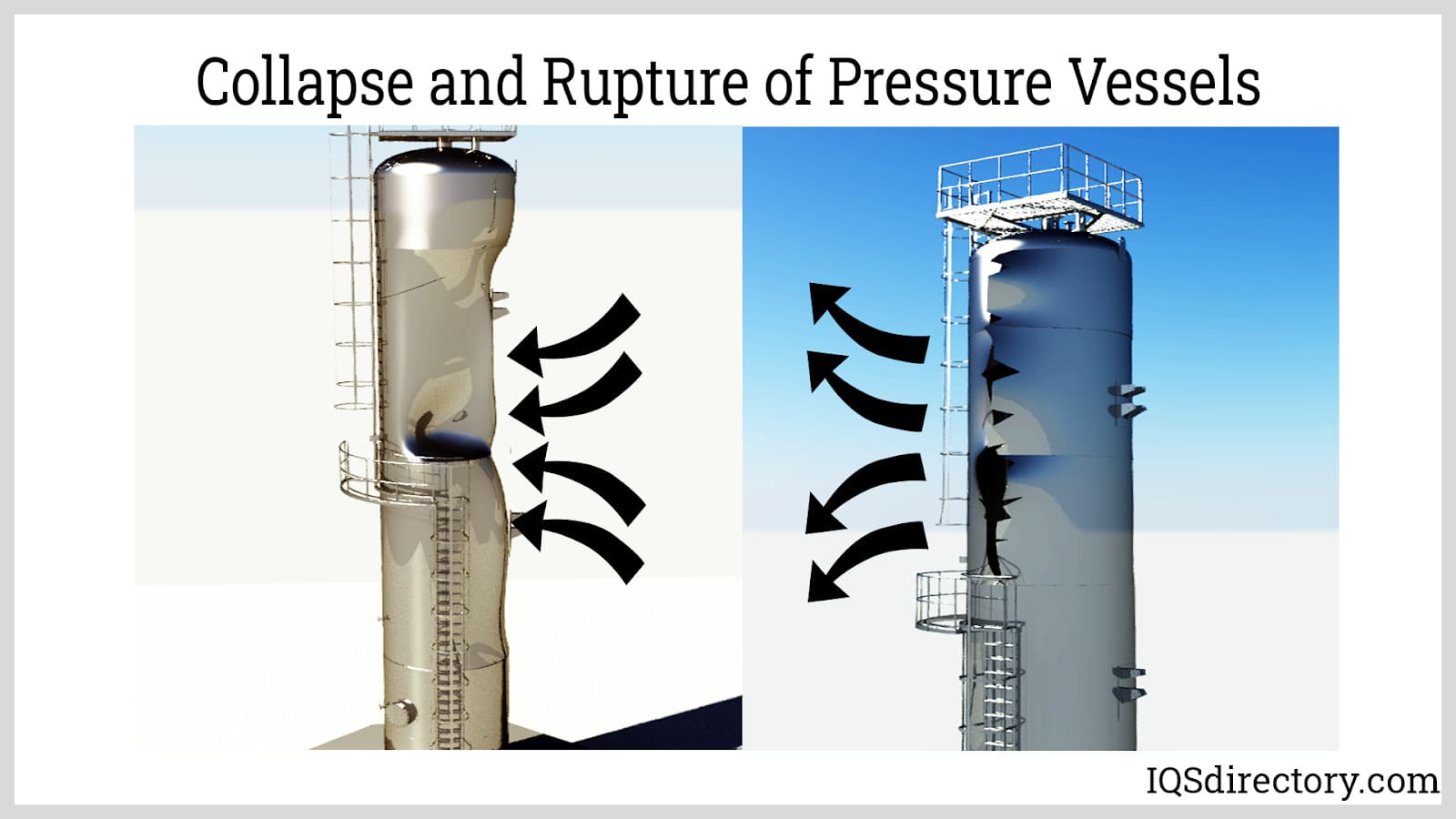
Pressure vessels are enclosed containers used to hold liquids, vapors, and gases at a pressure significantly higher or lower than the ambient pressure. They are widely used in various industries such as...
Please fill out the following form to submit a Request for Quote to any of the following companies listed on
Here is the most complete guide on the internet about
You will learn:
This section will explore what pressure tanks are, how they are manufactured, and the way they function in various applications.
Pressure tanks are vessels intended to store, regulate, and transport gases, vapors, or liquids under conditions where the pressure is higher than atmospheric levels, often referred to as elevated pressures.
Pressure vessels are sealed containers used to store liquids, vapors, and gasses at much higher or lower pressures than the surrounding atmosphere. They are widely utilized in a variety of industries, including petrochemical, gas and oil, chemical, and food processing. Pressure vessels include items such as reactors, flash drums, separators, tanks, and heat exchangers.
Pressure vessels need to function within specified temperature and pressure thresholds, referred to as their safety limits. Their design, fabrication, and inspection are carried out by certified experts and adhere to strict regulations to prevent accidental releases or leaks that could pose risks to the environment. Key standards for these vessels include the API 510 Pressure Vessel Inspection Code and ASME Section 8 of the Boiler and Pressure Vessel Code.
The key difference between pressure tanks and pressure vessels is their maximum allowable operating pressure (MAOP). Pressure vessels are built to endure pressures well beyond atmospheric levels, frequently exceeding 15 PSI, with MAOPs that can reach up to 3000 PSI or higher in certain cases. On the other hand, pressure tanks are used for the static storage of liquids and usually have an MAOP of 15 PSI or less, often functioning at or below atmospheric pressure.
The design and safety criteria for pressure vessels are regulated by ASME Section VIII, encompassing Divisions 1, 2, and 3. In Europe, equivalent safety standards are set by BS5500 and other national regulations. Pressure tanks are generally designed to adhere to industry-specific standards and pressure limits as detailed below:
In conclusion, pressure vessels are usually sealed units, while pressure tanks can be either open or closed, especially in vertical cylindrical configurations. For instance, a fuel tank may have an open top with a breather to balance pressure with the surrounding atmosphere. Moreover, pressure tanks are generally larger than pressure vessels, and the maintenance of pressure vessels can be riskier due to the increased potential for explosions.
The manufacturing process of pressure tanks involves these key steps:
Pressure tanks are commonly made from steel and stainless steel. Steel is chosen for its robustness, strength, and resilience under significant stress and extreme temperatures. Stainless steel provides these advantages as well as being easy to sanitize and resistant to corrosion, which makes it ideal for use in sanitary environments like food and beverage processing, medical applications, or laboratory settings.
In addition to steel and stainless steel, pressure tanks can be made from materials such as aluminum, carbon fiber, titanium, zirconium, and various high-strength polymers. Depending on the application requirements, pressure tanks may also be coated with polymers, rubber, metals, or ceramic materials to improve structural strength and prevent leaks.
When choosing a material, the following factors should be taken into account:
The design calculations for pressure vessels involve several key parameters. These factors are crucial for calculating the thickness of the shell and head walls.
Design Pressure: This parameter is essential for defining the specifications of the vessel. It is determined based on the maximum operating pressure, accounting for pressure variations during start-ups, emergency shutdowns, and process fluctuations. The design pressure must always exceed the system's maximum operating pressure and impacts the vessel's pressure release mechanisms to prevent explosion risks. It is generally recommended that the design pressure be 5-10% higher than the peak operating pressure. For vessels that may be exposed to vacuum conditions, the design pressure should be specified to handle a complete vacuum (-14.7 psi).
Maximum Allowable Working Pressure (MAWP): MAWP is the maximum pressure at which the vessel is designed to function safely at its designated temperature. It is the highest pressure that the weakest part of the vessel can handle at this temperature. Established by the American Society of Mechanical Engineers (ASME), MAWP is crucial for ensuring safety by preventing operation above this threshold, thus reducing the risk of explosions. Unlike design pressure, which accounts for operational conditions, MAWP is a more general measurement based on the vessel's material limits. Factors such as corrosion and wear can reduce the MAWP, while the design pressure is determined by operational conditions and may be equal to or lower than the MAWP.
Design Temperature: Temperature impacts the maximum allowable stress on a vessel, as material strength diminishes with higher temperatures and becomes brittle at very low temperatures. To calculate the maximum allowable pressure, the vessel must not be operated at temperatures that exceed the design temperature. This design temperature is set higher than the maximum operating temperature but lower than the minimum temperature the vessel could experience.
When determining the design temperature, several guidelines should be followed. It is recommended to set the design temperature 500 degrees Fahrenheit above the highest operating temperature and 250 degrees Fahrenheit below the lowest operating temperature. For vessels operating within the temperature range of -30°C to 345°C, a maximum design temperature allowance of 250°C is suggested. Additionally, designers should consider any potential disturbances that could significantly impact the temperature of the pressure tank.
Maximum Tolerated Stress: Maximum tolerated stress is calculated by applying a safety factor to the maximum allowable stress. This safety factor compensates for possible deviations from ideal conditions in the construction and operation of the pressure vessel.
Joint Effectiveness: The ASME Boiler and Pressure Vessel (BPV) Code classifies welded joints into four categories. Joint effectiveness measures the ratio of the base material's strength to that of the welded joint. Typically, the strength of welded joints is lower. In the absence of additional inspection and radiographic testing, welded joints are regarded as weaker due to potential defects like porosity. The ASME BPV Code Section offers guidelines for assessing joint efficiency.
Corrosion Allowance: The estimation of corrosion allowance follows various guidelines that can differ by manufacturer. Generally, a corrosion allowance ranges from 1.5 to 5 mm. It is advisable to allocate 0.25 to 0.38 mm per year, or approximately 3 mm over a decade. For environments with high corrosion rates, allowances can reach up to 8.9 mm, whereas for less corrosive environments, around 3.8 mm is typical. Air receivers and steam drums often have a corrosion allowance of 1.5 mm. In heat exchanger systems, where wall thickness impacts heat transfer efficiency, the corrosion allowance should be minimized.
The shell and heads of the vessel are manufactured through forging, rolling, and welding metal plates. The thickness of these plates, which determines the wall thickness, is carefully calculated according to the previously discussed criteria. Following this, various auxiliary equipment, devices, and accessories are installed to ensure the proper functioning of the pressure vessel.
Post-weld heat treatment is used to relieve stresses that develop during the welding and forming processes.
Pressurized containment vessels can be built to comply with the standards established by the American Society of Mechanical Engineers (ASME). Often referred to as ASME tanks, these vessels are designed to meet ASME’s rigorous criteria for design, construction, fabrication, operation, and maintenance. To maintain ASME certification, these pressure tanks must undergo regular inspections. While ASME certification is not always required, many manufacturers adhere to these standards to guarantee quality and safety for their customers.
Pressure tanks can also conform to a range of local and international standards, including those set by UL, ASTM International, TUV, USDA, ISO, Canada, and the European Union, among others. Additionally, many manufacturers produce vessels that do not adhere to specific codes. For the latest and most relevant information on requirements and options tailored to a customer's needs, it is recommended to consult with an experienced pressure tank manufacturer.
Pressure vessels are designed to function at specific pressure levels required for their intended uses, such as storing air in scuba tanks. They manage pressure either directly through valves and gauges or indirectly via heat transfer. Pressure levels can vary significantly, from 15 psi to 150,000 psi, with temperatures freq
Key factors to consider when selecting pressure tanks include:
Material selection is critical in pressure tank manufacturing and greatly affects the cost. Options include carbon and low alloy steels, nickel alloys such as Monel, Inconel, and Hastelloy, various stainless steels including series and high-temperature grades, duplex stainless steels like 2205 and 2507, and aluminum. The choice of material depends on the specific process conditions and the tank's application, making it essential to select the most appropriate material for the tank's intended use and requirements.
Design is a critical factor in developing a new pressure tank, as it must withstand challenging industrial conditions. The weld construction is particularly important, as defects can lead to significant failures. BEPeterson guarantees that all vessels are designed to meet ASME standards using the latest version of Compress software. Advanced fabrication techniques are utilized to refine designs, ensuring that cost considerations are thoroughly addressed.
When acquiring a pressure tank, it is crucial to verify that it adheres to the applicable industry standards and specifications:
Manufacturers perform a range of tests to evaluate the tank's load-bearing capacity and performance under extreme conditions. These tests include Ferrite Testing, Hydro-testing, Positive Material Identification (PMI), Helium Leak Testing, Dimensional Inspection with a FaroArm, as well as Radiography and Non-Destructive Examination (NDE) methods such as Penetrant Testing (PT), Ultrasonic Testing (UT), and Magnetic Particle Testing (MT). These assessments offer valuable insights into the tank’s strength and dependability.
This chapter will explore the various classes and types of pressure tanks.
This tank ensures a steady pressure in a well water system. In a residential well system, a pump—either submersible or jet—pulls water from the ground into the home. The well pressure tank also acts as a storage reservoir, holding water for the household and triggering the pump when the water level drops and needs replenishing. Below, three types of pressure tanks in this category are examined.
Commonly known as galvanized or stainless steel pressure tanks, these units store both water and air together. They usually have an interior coating to prevent corrosion, often referred to as "glass-lined" or "epoxy-coated."
In this type of tank, air and water are not separated. Although less common today, these tanks are still used. Compressed air is employed to apply pressure to the water, achieving the necessary pressure for your home. While these tanks are often larger than other types, their capacity can be comparable to that of smaller tanks. This can result in the pump cycling on and off more frequently, which may lead to premature wear and failure. Additionally, these tanks are susceptible to water-logging due to air loss through corroded pinholes.
These tanks feature two distinct compartments: one for air and one for water. A rubber diaphragm, which is permanently affixed inside the tank, separates these compartments and adjusts with the water level.
As water is pumped into the lower compartment, it pushes the diaphragm upward into the air chamber. This movement triggers a switch that turns off the pump.
While this system is effective, it has some potential drawbacks. One issue is the possibility of the diaphragm detaching from the tank’s interior, which allows water to enter the air chamber. If this happens, the tank will need to be replaced. Another concern is when the diaphragm folds over itself, leading to inaccurate readings and potentially causing premature pump burnout and tank failure.
These tanks feature two separate chambers for air and water. The bladder, resembling a large balloon filled with air, expands and contracts to activate the pump. Unlike single-chamber or diaphragm tanks, bladder tanks are self-contained and avoid the issues associated with those types. Moreover, since air and water are kept apart, there is no mixing, which eliminates the need for additional air. Bladder tanks also generally have a longer lifespan compared to diaphragm tanks.
A steam drum is an essential component of a water-tube boiler, located at the top of the water tubes. It serves as a reservoir for both water and steam, collecting the steam produced by the water tubes and separating it from the water. The density difference between hot and cold water facilitates the accumulation of the hotter water. Consequently, the steam within the steam drum becomes saturated.
The steam collected from the top of the drum is distributed to various processes. To produce superheated steam, the saturated steam is further heated, typically for use in steam turbines. Saturated steam is drawn from the drum's upper part and directed through a superheater before it returns to the furnace. A mixture of water and steam enters the steam drum through riser tubes, where a demister removes water droplets to produce dry steam. The steam drum is equipped with a safety valve, a water level indicator, and a level controller. Additionally, feed water is supplied to the steam drum through a dedicated feed pipe running along its length.
A steam drum can be utilized either independently or in conjunction with a mud drum or feed water drum positioned at a lower level. Boilers that feature both a steam drum and a mud or feed water drum are known as bi-drum boilers, whereas those with just a steam drum are referred to as mono-drum boilers. Generally, bi-drum boilers are designed for lower pressure applications, while mono-drum boilers are intended for higher pressure applications.
Steam drums come in various types, including:
Three and Four Drum Boilers: While these designs are traditional, they continue to be utilized in various industries today.
Bi Drum Boilers: These boilers are utilized for power and steam generation but have largely been replaced by single drum boilers in power generation due to their lack of reheating capability. Single drum boilers are favored for their efficiency in plants with high heat rates. Despite this, bi drum boilers are still widely used in process steam generation due to their capacity to manage significant load variations and adapt to changing demands.
Single Drum Boilers: These boilers are predominantly used in power plants for generating electricity. They are capable of handling higher pressure limits than bi drum boilers due to reduced stress concentration. Single drum boilers feature a single drum with welded downcomers and are suitable for both reheat and non-reheat applications. They can be designed as corner tube boilers, where the downcomers create the structural framework, or as top-supported boilers, where the assembly is supported by an external frame and the top drum.
Storing ammonia is essential in many industries. Given its unique properties, ammonia typically needs to be kept in either high-pressure storage tanks or low-temperature refrigerated vessels. Managing substantial quantities of ammonia involves tackling several issues related to safety, health, and environmental impact.
Low-pressure ammonia storage is becoming more prevalent for two key reasons. Firstly, it involves a much lower capital expenditure per volume unit. Secondly, it provides enhanced safety compared to spherical storage systems, which operate at pressures exceeding atmospheric levels. Due to the widespread industrial production of ammonia, storing it at atmospheric pressure and -33°C has become a common standard.
For managing large volumes, such as up to 50,000 tonnes of ammonia, on-site storage tanks are utilized, maintaining a temperature of -33°C.
Known by various names including hot water storage tank, thermal storage tank, unit thermal storage tank, hot water cylinder, or heat storage tank, this device is used to store hot water for applications like space heating or domestic use.
Because of its large specific heat capacity, water is an excellent heat storage medium. This means that it can mass more heat per unit weight than other substances. Water is inexpensive and non-toxic. A well-insulated tank can keep stored heat for days, lowering fuel expenses. Hot water tanks may have a gas or oil burner system as well as electric immersion heaters. Some employ an external heat exchanger, such as a central heating system or warm water from another energy source. In the household environment, the most common are an electric immersion element, fossil-fuel burner, or a district heating scheme.
Water heaters designed for tasks such as washing laundry or bathing are equipped with thermostat controls that allow temperature settings ranging from 40 to 60°C (104 to 140°F). These heaters are connected to the home's cold water supply.
If the water in the tank has a high mineral content, such as limestone, boiling can lead to mineral buildup or scaling. Over time, this can cause leaks due to corrosion, a problem exacerbated by dissolved oxygen in the water, which accelerates the corrosion of both the tank and its fittings.
A process tank is a container or vessel used for mixing or batching chemicals, wastewater, feeds, or other substances, or for preparing these components to achieve a specific outcome. The term encompasses all related piping and fixtures necessary for the tank's intended function.
This chapter will explore the various uses and advantages of pressure tanks.
Pressure tanks are utilized across various industries for storing and transporting substances. These industries include food and beverage, chemicals, recreation, plastics and polymers, rubber, military and defense, water treatment and filtration, pharmaceuticals, oil, fuel, and energy.
Furthermore, they are frequently associated with well systems, from which residential residences and structures obtain their water supply. Other pressure tank applications include nuclear reactor vessel operation, autoclaves, recompression chambers, and road vehicle air brake reservoirs, powering of air tools, and the functionality of diving cylinders and other cylinders of gas utilized in medical, chemical, and industrial processes. The most common pressure tank applications are discussed below.
In the oil and gas industry, pressure vessels are widely used as receivers for high-temperature and high-pressure physical and chemical processes. These vessels serve various functions, but their construction generally follows similar principles. For example, distillation columns are utilized to separate feed streams into different components based on their boiling points. Due to the similarities in their manufacturing processes, pressure vessels and columns are often produced by the same manufacturers.
Stainless steel and carbon steel are the two most often utilized building materials in the oil and gas industry. Other components, in addition to the external body, are required for a pressure vessel to be usable, like vessel internals and distillation trays. Such components are extremely complicated, necessitating standards that differ greatly from those required for the manufacturing of pressure vessels, which are provided by specialized vendors.
This type of pressure tank is used for processes such as chemical reactions, which result in significant transformations of the contents within the container. These operations might involve combining substances to create a new product, breaking down a product into one or more new products, or removing components from an existing product to produce a different item. In the chemical industry, various types of pressure vessels are often utilized simultaneously to achieve these processes.
Pressure tanks are essential in the energy sector for several reasons. They play a crucial role in managing hazardous gases, which are often stored in facilities such as oil refineries and metal works. Additionally, nuclear power plants employ specialized pressure vessels known as Reactor Pressure Vessels (RPVs). These large cylindrical steel containers house the reactor core, steam, and cooling water. RPVs must be extremely reliable to withstand high temperatures, pressures, and neutron irradiation, making them a critical component in a nuclear power plant. It is important to note, however, that not all power reactors use reactor pressure vessels.
Pressure tanks are utilized across a range of industrial applications, including nuclear reactors and mining operations. They also play a role in everyday processes such as heating water and distillation. These tanks are essential for safely storing liquid gases and are crucial for handling and transporting volatile chemicals like propane, ammonia, and LPG.
In water distribution systems, pressure tanks provide notable benefits, including extending pump lifespan and reducing hydraulic shocks when the pump is activated. As water is pumped into the tank, it compresses the air inside, which helps manage pressure more efficiently and protects the system from sudden pressure changes.
Elastic Deformation - Elastic buckling or instability is influenced by factors such as the vessel's shape, stiffness, and material properties. These factors collectively determine the vessel's resistance to buckling under applied loads.
Brittle Fracture - Brittle fractures can occur at low or moderate temperatures and are often observed in vessels made of low carbon steel. These fractures may be detected during hydrotesting in the 40° – 50°F range, especially where minor flaws are present.
Excessive Plastic Deformation - To prevent excessive plastic deformation and gradual collapse, the ASME's main and secondary stress limits are established. These limits ensure that the vessel maintains its structural integrity under operating conditions.
Stress Rupture - This phenomenon involves progressive fracture due to creep deformation, which is a time-dependent process, or fatigue resulting from cyclic loading. Creep and fatigue are distinct but can both lead to stress rupture in pressure vessels.
Plastic Instability - Also known as "incremental collapse," this phenomenon occurs due to cumulative cyclic strain or deformation. It leads to vessel instability caused by plastic deformation over time.
Stress Corrosion - Chlorides can induce stress corrosion cracking in stainless steels, whereas caustic environments may cause stress corrosion in carbon steels. Selecting the appropriate materials is crucial for preventing these issues.
High Strain - Low cyclic fatigue, often influenced by strain, is typically observed in materials with low strength and high ductility.
Despite these drawbacks, the benefits of using pressure tanks far outweigh the potential issues.
Pressure vessel closures are pressure-retaining devices that allow easy access to pipelines, pressure vessels, pig traps, filters, and filtration systems. Pressure vessel closures often enable maintenance staff access. An elliptical access hole form is typically utilized, allowing the closure to be inserted through the opening and twisted into the working position, and is kept in place by an outer bar secured by a central bolt. Internal pressure prevents it from being opened accidentally while under load.
Pressure tanks are vessels that are used to store or convey gasses, vapors, and fluids at pressures greater than atmospheric pressure, also known as high pressures. They come from pressure vessels which are sealed containers that store the same contents. Steel and stainless steel are the ideal materials for pressure tanks. Material selection, design industry standard, and pressure tests conducted are to be considered when choosing a pressure tank. Several various types of tanks may be considered as pressure tanks. These examples are hot water tanks, ammonia tanks, steam drums, process tanks, and refractory lined vessels.
Pressure vessels are enclosed containers used to hold liquids, vapors, and gases at a pressure significantly higher or lower than the ambient pressure. They are widely used in various industries such as...
Stainless steel tanks are widely used in food, beverage, dairy, medicine, cosmetics, and other manufacturing processes where cleanliness and purity are important. These are also used in industrial plants for storing chemicals and gases where strong resistance from chemical degradation is required...