Temperature Sensors
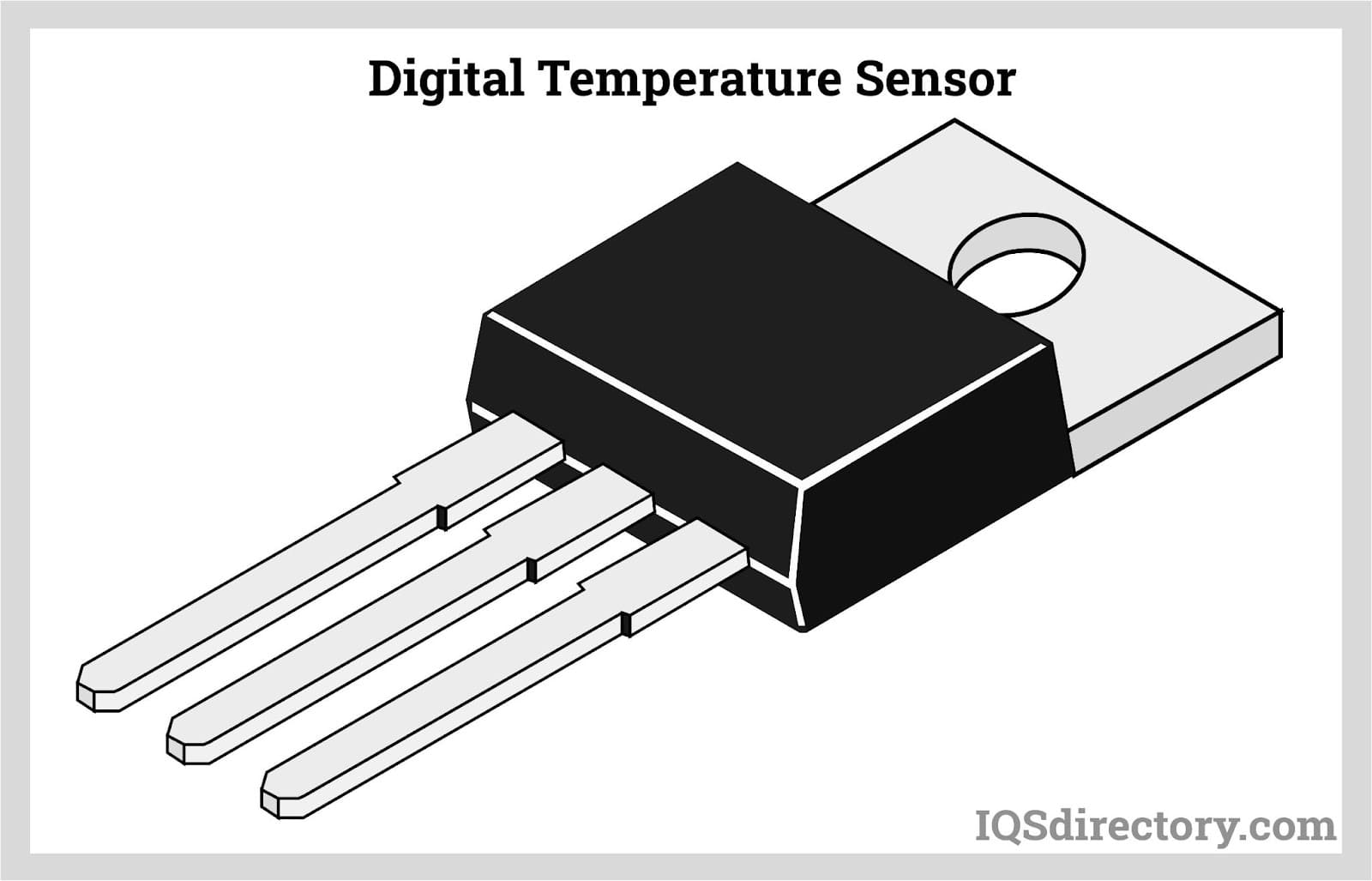
Temperature sensors are devices that detect and measure coolness and hotness and convert it into an electrical signal. Temperature sensors are utilized in our daily lives, be it in the form of domestic water heaters...
Please fill out the following form to submit a Request for Quote to any of the following companies listed on
This article contains everything you need to know about RTD sensors.
You will learn more about topics such as:
A Resistance Temperature Detector (RTD) is a non-active temperature measurement device that operates on the idea that a metal's resistance changes with temperature variation. The electrical current traversing the sensor's element or resistor produces a resistance value, which is then recorded by a connected instrument. This instrument interprets the resistance measurement into a temperature reading according to the specific resistance characteristics of the RTD sensor.
As the temperature of the metal increases, its electrical resistance experiences a corresponding rise. RTD sensors are engineered to assess temperature by identifying the predictable resistance fluctuation as the material's temperature changes. These sensors are highly regarded for their precision, reliability, and durable stability in temperature measurement applications.
RTD sensors are manufactured using a variety of element types, each adhering to different standards and covering various temperature ranges. They come in various sizes and accuracy levels, but all share the basic function of measuring temperature through changes in resistance, with each type having a specific resistance value for its temperature range.
Resistance elements are the core component of an RTD and are too delicate and sensitive to be used in their raw form. Thus, they require protection and shielding. RTD readings are expressed in Ohms (Ω), which measures electrical resistance.
Different metals are used as resistance elements, each providing resistance measurements across different temperature ranges.
The sensing component of an RTD sensor is its element, which changes resistance with temperature variations. Platinum is the most commonly used element, though other metals such as copper, nickel, tungsten, Balco, and iridium are also employed.
Platinum sensor elements are constructed from pure platinum wire and feature a positive temperature coefficient. Their linearity and long-term stability make platinum RTD elements highly accurate for industrial applications. They can use copper wire extension leads and are ideal for industrial settings that demand stability and linearity across a broad temperature range.
Nickel elements have a more limited temperature range compared to platinum, as their resistance changes significantly with temperature and becomes non-linear above 300°C (572°F), requiring error correction for accurate temperature measurement. Although they offer good corrosion resistance and are less expensive than platinum RTDs, nickel elements age quickly and lose accuracy over time. Their effective temperature range is from -80°C to 260°C (-112°F to 500°F).
Copper offers good linear resistance with temperature changes, but its low resistivity requires a longer element compared to platinum. Due to copper's tendency to oxidize, its use is limited to temperatures below 150°C (302°F). Copper RTDs are primarily used for measuring winding temperatures in motors, generators, and turbines.
While copper elements are relatively inexpensive and exhibit good linearity, they quickly lose their linearity and drift, which can affect temperature accuracy. They are best suited for applications in non-oxidizing environments where their linearity and cost benefits outweigh their limitations.
The chart below provides a comparison of the performance of copper, nickel, and platinum elements.
Effectiveness of Copper, Nickel, and Platinum Elements | |||||||||
---|---|---|---|---|---|---|---|---|---|
RTD Type | Maximum Measurement Range | Long Term Stability | Corrosion Resistance | Temperature Vs. Resistance Linearity | Typical Resistance At 0°C | Typical Resistance At 100°C | Change In Resistance Resistance Ratio 0... 100°C | (R100.Ro) / Ro | Alpha, (α) (R100..Ro) /(100 x R0) |
Platinum | -200... 850°C | Excellent | Excellent | Good | 100Ω | 138.5Ω | 38.5Ω | 0,385 | 0,00385 |
Nickel | -80... 260°C | Fair | Good | Fair | 120Ω | 200.64Ω | 80.64Ω | 0,672 | 0,00672 |
Copper | -200... 260°C | Good | Fair | Excellent | 9.035Ω | 12.897Ω | 3.86Ω | 0,427 | 0,00427 |
Balco sensors are made from an annealed resistance alloy composed of 70% nickel and 30% iron. A Balco 500 ohm sensor offers relatively linear resistance within the temperature range of -40°C to 116°C (-40°F to 240°F). Balco has similar thermal conductivity to nickel but with twice the resistivity. Like copper, Balco RTD sensors are cost-effective and feature a high resistance coefficient, excellent linearity, strong mechanical properties, and some corrosion resistance.
Tungsten is known for its high resistivity, making it suitable for high-temperature applications. However, tungsten is brittle and challenging to work with and shape.
All metals exhibit an increase in resistance with a rise in temperature. However, some metals have better resistivity, making them more suitable for RTD sensors. Metals like gold and silver are rarely used for RTD sensors due to their low resistivity.
When evaluating a metal for RTD applications, purity is a crucial factor, as it significantly impacts the metal's resistivity. To specify and select elements for RTD use, their resistance in ohms (Ω) is measured at zero degrees Celsius, ideally resulting in 100 Ω of resistance.
The operating principle of an RTD sensor is straightforward. As the temperature of the metal increases, its resistivity changes. This change in resistance can be measured and used to determine the temperature.
RTD sensors are crucial in manufacturing and sensitive temperature processes due to their high accuracy and resistance to temperature fluctuations, vibrations, and shocks. They operate with a limited DC current to prevent overheating and ensure reliable performance.
When placing the sensor into an application, it is recommended to have a thermowell, a closed end tube mounted on the process stream. The process transfers heat to the thermowell wall and on to the sensor. A thermowell is a thermal conductive protrusion placed in a process line that allows the placement of sensing devices without needing to open a hole in the line.
Most RTD sensors are encased in a protective sheath made of stainless steel or Inconel, which shields the sensing element from environmental factors and mechanical damage. This design allows the sensor's measuring end to be inserted directly into the measurement area, while termination wires connect the sensor to the recording device.
When installing the sensor, ensure that the cables at the connecting head remain straight and untwisted while threading the sensor into place. Keeping the wires disconnected during insertion can prevent issues with the cables.
For accurate calibration, all connecting wires should be of the same size and length. The insertion depth of the sensor should be ten times the diameter of the stem.
The diagram below illustrates an RTD sensor inserted using a thermowell.
The frequency of RTD calibration depends on factors such as temperature cycles, vibrations, and shock. Typically, the user determines how often calibration is needed. Calibration is performed by comparing the sensor's resistance to a known working standard. Ideally, calibration should be conducted with the sensor in its operational position.
Even though most sensors are protected by a sheath or installed in a thermowell, regular maintenance involves checking for damage from corrosion, shock, vibrations, or other factors. Sensors that show signs of damage should be replaced.
RTD sensors are classified based on the construction of their temperature sensing elements. Two common types are thin film and wire wound. The choice of RTD sensor depends on the specific environment and application in which it will be used.
The use of resistance temperature sensors began in the middle of the first industrial revolution, and they were assembled using copper wire and a galvanometer. Copper wire was replaced by platinum when it was discovered that platinum could measure a wider range of temperatures.
RTD sensors are classified according to their design tolerances as specified by the International Standard IEC 751. These classifications include Class A, Class B, and Class C, with the DIN curve being the most commonly used standard. The DIN curve defines the resistance vs. temperature characteristics for a platinum, 100 ohm sensor, including standardized tolerances and a specified temperature range.
Manufacturers provide detailed descriptions and designations of their RTD sensors' tolerances in their catalogs, typically using the A and B classifications.
PARAMETER | IEC 751 Class A | IEC 751 Class B |
---|---|---|
R0 (Base Resistance) | 100Ω ± 0.06% | 100Ω ± 0.12% |
α (Alpha) | α = 0.00385±0.000063z/z/·C | α = 0.00385±0.000063z/z/·C |
Applicable Range | -200°C to +650°C | -200°C to +850°C |
Resistance Tolerance | ±(0.06 + 0.0008*|T| - 2*10-/*T2Ω (±0.06% at 0°C) | ±(0.12 + 0.0019*|T| - 6*10-/*T2Ω (±0.12% at 0°C) |
Temperature Deviation | ±(0.15 + 0.002*|T|)°C | ±(0.3 + 0.005*|T|)°C |
Thin film RTD elements consist of a thin metal layer deposited onto a ceramic substrate. This metal film is etched into a specific electrical circuit pattern to provide the desired resistance. Lead wires are then attached, and a protective coating is applied to both the substrate and the element. The image below shows a typical resistance pattern.
Thin film RTD sensors are known for their durability and reliability, offering resistance to shock and vibration. Their flat design allows them to be adapted for various applications, and they are available in a range of resistance types, tolerances, sizes, and shapes.
In the wire wound version of an RTD, a wire is coiled around the exterior of a ceramic or glass housing, known as a bobbin as illustrated in the diagram below. Glass core RTD sensors are suitable for immersion in liquids, while ceramic core RTD sensors are designed to measure extreme temperatures with high accuracy. Due to the specialized engineering and advanced manufacturing processes required, wire wound RTD sensors are typically more costly than thin film sensors.
Coiled RTD sensors feature a fine wire wound into a coil and housed within a ceramic or glass enclosure filled with a non-conductive powder. This design allows the resistance wire to expand and contract with temperature changes, reducing errors from mechanical strain. The surrounding powder enhances heat transfer, thereby improving the sensor's response time. Typically, the ceramic or glass housing is encased in a protective metal sheath for added durability.
The "Pt" in the PT100 designation signifies that the sensor uses a platinum element. The "100" refers to its resistance value of 100 Ω at 0°C (32°F). The PT100 RTD sensor is known for its high accuracy and stability, with minimal drift over time. Various PT100 sensors come with different temperature coefficients, represented by the Greek letter alpha (α), with the "385" coefficient being the most common.
The PT1000 is the second most commonly used resistance sensor, featuring a resistance of 1000 Ω. It is predominantly employed in two-wire applications. Known for its excellent accuracy and minimal drift over time, the PT1000 offers reduced lead wire distortion, which constitutes only a small fraction of the total resistance.
With its higher resistance value, the PT1000 requires less current, making it suitable for low-power configurations. This lower power consumption leads to reduced heat generation and fewer errors related to self-heating.
The two-wire RTD is the most straightforward circuit design. It features a single lead wire connected to each end of the element. The resistance in this setup is measured by accounting for the resistance in the lead wires and connectors, which can introduce some error or an overestimation of the actual temperature. This error can be corrected through calibration.
The three-wire configuration is commonly used in industrial applications. In this setup, two wires (A and B) connect to one end of the sensor and to the monitoring device, while the third wire (C) is connected directly to the element. All three wires are of equal length, ensuring that their resistances are equal. Despite its advantages, the three-wire configuration can still introduce errors that require calibration for accurate measurements.
The four-wire configuration, while the most complex and costly to install, offers the highest accuracy and precision. In this setup, DC current is supplied through two leads, A and C, while the voltage drop is measured by the remaining two leads, B and D. Since both the voltage drop and current are known, the resistance can be accurately determined, resulting in precise temperature readings across the system.
A variation of the four-wire design features two red wires connected to the element, with a white configuration looped around them. This design combines aspects of both the three-wire and four-wire methods.
RTDs and thermocouples are both used to measure temperature in Fahrenheit or Kelvin. They each convert temperature measurements into electrical signals, but they operate on different principles. RTDs rely on the change in electrical resistance with temperature, whereas thermocouples generate a voltage at the junction of two dissimilar metals, and this voltage varies with temperature changes.
Choosing between these sensors depends on their specific features rather than which one is universally better. Comparing their distinct attributes will provide a clearer understanding of their suitability for different applications.
Temperature measurements are often performed in challenging environments characterized by corrosive, oxidizing, and reducing conditions. Additionally, these settings may involve vibrations, noise, and electrical interference.
RTDs are constructed with wire wound elements housed in protective casings, making them durable and resistant to severe conditions. For enhanced protection, RTDs can be coated with perfluoroalkoxy (PFA) poly(tetrafluoroethylene), which is suitable for use in plating baths and high-pressure systems.
Thermocouples, encased in metal, are adept at handling corrosive and oxidizing environments. However, extra precautions should be taken when using exposed thermocouple junctions.
Thermocouples are more affordable compared to RTDs, which are priced two to three times higher than thermocouples while covering similar temperature ranges. The cost disparity arises because manufacturing thermocouples is less expensive. Additionally, thermocouples require periodic adjustments and calibrations and generally involve a longer setup and installation process.
RTDs can measure temperatures up to 1000°C, though achieving accurate readings above 400°C can be challenging. Thermocouples are capable of measuring temperatures up to 1800°C. Typically, RTDs are preferred for temperatures below 850°C, whereas thermocouples are more suitable for temperatures exceeding 850°C.
Most industrial processes operate within the range of 200°C to 400°C, making RTDs the optimal choice for these applications.
Both RTDs and thermocouples respond quickly to temperature changes, though thermocouples generally have a slightly faster response. RTDs can be adjusted to improve their response time.
The size difference between RTDs and thermocouples is minimal. Both instruments are compact, with diameters around 0.5 mm. It may be necessary to check the specific mounting location to ensure proper fit, although significant size differences are unlikely.
RTDs are more prone to failure in environments with significant vibrations due to their construction and design. In contrast, thermocouples are more resilient to vibrations and can provide reliable measurements in such conditions.
RTDs require a power supply and voltage to function. While the power needed is minimal, typically between 1mA and 10mA, it can cause the RTD’s platinum element to self-heat, potentially affecting accuracy. Thermocouples do not need a power supply and are unaffected by self-heating issues.
RTDs offer superior stability and can deliver consistent and precise readings over extended periods. Thermocouples, on the other hand, generate electromagnetic fields (EMFs) that can alter due to oxidation, corrosion, and changes in the sensing elements' metallurgical properties. Once a thermocouple starts drifting, it cannot be reversed.
Thermocouples can measure temperatures very precisely at the exact point where the two metals are joined, known as the “naked tip”. RTD sensors provide an average temperature reading across the entire platinum element, which can be a limitation for larger elements like the PT100. However, smaller elements such as the PT1000, which can be as small as 1 mm x 1.5 mm, experience fewer issues.
RTD sensors generally exhibit less drift, enabling them to provide stable readings for longer periods compared to thermocouples. Thermocouples tend to experience significant drift due to factors like inhomogeneous conductor wires, exposure to heat and chemicals, or mechanical damage like bending or squeezing. Consequently, thermocouples need more frequent recalibrations and adjustments.
Thermocouple sensors react quickly to changes at their contact point, the naked tip, but take longer to achieve thermal equilibrium due to cold junction compensation that does not respond as swiftly as the hot junction. RTD sensors are more robust and faster in reacting to temperature variations.
RTDs are generally more accurate for industrial applications, with precision up to 0.1°C, whereas thermocouples typically have an accuracy of around 1°C.
The chart below offers a brief comparative tool for examining RTDs, thermocouples, and thermistors.
Temperature Comparison Chart | |||
---|---|---|---|
RTD | Thermocouple | Thermistor | |
Temperature Range | -260 - 850°C (-436 - 1562°F) | -270 - 1800°C (-454 - 3272°F) | -80 - 150°C (-112 - 302°F) |
Sensor Cost | Moderate | Low | Low |
System Cost | Moderate | High | Moderate |
Stability | Best | Low | Moderate |
Sensitvity | Moderate | Low | Best |
Linearity | Best | Moderate | Poor |
Specific for | -General Purpose Sensing -Highest Accuracy -Temperature Average |
-Highest Temperatures | -Best Sensitivity -Narrow Ranges (e.g. medical) -Point Sensing |
For applications needing high accuracy and operating at temperatures below 500°C, RTD temperature sensors are ideal. Thermocouples, which offer a broader temperature range, are better suited for processes that require rapid response times and precise point measurements.
RTD sensors provide superior accuracy, stability, and repeatability with minimal drift. They offer a strong output signal with enhanced sensitivity and linearity. However, RTDs are more costly, have a narrower operational range, and lower maximum temperature limits. On the other hand, thermocouples are more affordable, robust, and capable of measuring a broader temperature range but tend to have lower accuracy and greater drift, necessitating frequent recalibrations, which can increase their overall cost.
When selecting a temperature sensor, it's crucial to weigh the benefits and drawbacks of RTDs and thermocouples. Key factors include their specific functions and the characteristics of the monitored process. Additionally, for applications where space is a concern, it’s important to note that RTDs are generally larger compared to thermocouples.
Thermocouples come in different types, each designed for particular temperature ranges and applications. Each type is built to suit specific environmental conditions and operational needs.
The various types of thermocouples are:
Sensors play a crucial role in manufacturing by measuring physical phenomena through the properties of metals and fluids. Among these, the resistance temperature detector (RTD) stands out as a precise, robust, and reliable instrument that provides valuable data for application monitoring.
The use of RTD sensors has grown due to their predictable linear resistance change with temperature, which ensures consistent and accurate temperature readings.
In the automotive sector, RTD sensors are extensively employed to gauge engine temperature, air temperature, external temperature, and fluid levels. Their advantages in this field include their non-heating properties and flexibility, making them ideal for various automotive applications.
For solar power systems, uniform heat distribution is crucial for efficient electricity generation. RTD sensors, which do not overheat, are well-suited for monitoring temperatures in solar panels. They also serve a similar purpose in grid-connected wind turbines, where they help track temperature variations.
In drug manufacturing, precise temperature control is essential to prevent damage to formulations. RTD sensors are integral to research, formulation, testing, and production processes, where they ensure accurate temperature regulation in a highly controlled environment.
Similar to the pharmaceutical industry, the chemical sector demands stringent temperature control. RTD sensors are used in various specialized chambers and systems to maintain the accuracy and safety of chemical processes and experiments.
The semiconductor industry requires advanced temperature control and heating solutions. RTD sensors are engineered to meet the rigorous demands of wafer processing, providing the necessary accuracy, repeatability, and stability in high-temperature conditions.
The applications of RTD sensors extend beyond the mentioned industries, highlighting their importance across various sectors.
Temperature monitoring is essential in every stage of food production, including manufacturing, storage, and shipping. RTD sensors are utilized to ensure proper temperature control throughout these processes.
In heating, ventilation, and air conditioning systems, RTD sensors are used for temperature monitoring, fire detection, and climate regulation.
In aerospace, RTD sensors serve similar functions as in automotive applications, monitoring engine temperatures, coolant systems, compressors, fuel tanks, and fire control mechanisms.
Temperature monitoring is critical for machinery, electric motors, windings, generators, ovens, and microwave power systems to prevent damage and ensure safe operation.
In the medical field, precise temperature control is vital for patient care, including in infant incubators, respiratory devices, and dialysis machines.
In communication systems, RTD sensors help manage the heat generated by amplifiers and transmitters, which is crucial for maintaining optimal performance.
RTD sensors are widely used in consumer products to regulate temperature. They can be found in everyday items like coffee makers, cell phones, washing machines, and electric blankets, ensuring consistent and safe temperature management.
Resistance temperature detectors (RTDs) consist of six primary components: the sensing element, wiring, protective tubing, connection fitting, outer diameter, and termination. The arrangement and specifications of these components define the different types of RTD sensors, influencing their accuracy and performance. Despite variations in capabilities, the fundamental components remain consistent across RTD sensors.
Typically, an RTD features a sensing element made from platinum, nickel, or copper. These metals are chosen for their accurate and positive temperature coefficient. Among them, platinum is the most prevalent due to its superior resistance to corrosion and long-term stability.
All metals exhibit resistance changes with temperature fluctuations. RTD sensor elements are selected for their consistent linear response to temperature variations. Initially, copper was used in RTD sensors, but it was later found that platinum provided superior performance and more precise measurements.
Contemporary RTD sensors are typically manufactured using copper, nickel, and platinum. Additionally, Balco, a nickel alloy, is employed due to its cost-effectiveness.
Copper is widely used for wire leads in two, three, or four wire RTD configurations. These wires are typically insulated with materials such as fiberglass, Teflon, or various plastics. The wire leads must be of precise length to ensure proper resistance matching for the RTD and to establish a connection with the reading device.
Stainless steel and Inconel are commonly used tubing materials for RTD sensors. Stainless steel is suitable for applications with temperatures up to 500°C or 260°F. For temperatures exceeding these limits, Inconel is preferred. The choice of tubing material should ensure that it complements the RTD sensor's durability and is suitable for the various environmental conditions where the sensor will be deployed.
The connection fitting is essential for securely attaching the RTD sensor to the application and integrates with fittings used for other temperature measurement devices. Brass and stainless steel are two metals frequently used for these fittings. Brass is selected for its resistance to corrosion, while stainless steel offers both corrosion and chemical resistance. The design of these fittings ensures straightforward sensor installation and proper alignment of wire leads, preventing twisting or crimping.
RTD sensors are available in various outer diameters, typically ranging from 0.063 inches (1.6 mm) to 0.5 inches (12.7 mm).
The termination establishes the connection between the RTD sensor and the monitoring device. This can be achieved through various methods such as soldering or crimping. Terminations at the cold end may include options like bare wires, as well as various types of plugs and jacks.
Lead wires can introduce minor errors in RTD sensor calibration by adding extra resistance that is not part of the measured environment but affects the temperature coefficient. Several methods are employed to address this issue and mitigate its impact.
In critical applications demanding high accuracy and precision, it is crucial to implement measures to prevent calibration errors caused by lead wires.
Since their inception during the first industrial revolution, RTD sensors have become integral to manufacturing processes. Their exceptional accuracy and stability make them ideal for temperature monitoring, delivering instant data with minimal effort.
In industrial operations, precise temperature monitoring is essential to ensure the proper functioning of equipment. RTD sensors provide a reliable means to achieve and maintain optimal temperatures due to their precision and accuracy.
RTD sensors are generally more costly compared to other temperature measurement methods. However, the investment is justified by their accuracy, longevity, repeatability, and stability, leading to overall cost savings.
One of the key requirements for a sensor is its ability to deliver data promptly. RTD sensors offer quick and precise temperature readings. Advances in thin film Pt100 sensors have significantly enhanced their response times.
RTD sensors are favored for their accuracy, with deviations typically within 0.1°C. This high level of precision is attributed to their linearity, which ensures consistent performance.
Linearity refers to a sensor's capability to respond proportionally to temperature changes across its entire range. A sensor with high linearity provides a resistance change that accurately reflects the temperature change, contributing to the RTD sensor’s renowned accuracy and reliability.
Stability is defined by a sensor’s ability to deliver consistent and precise readings over an extended period. RTD sensors maintain stable and repeatable readings longer than other temperature sensors. Sensor stability is assessed based on drift and linearity.
RTD sensors are constructed with high-quality pure metals, which are crucial for optimal performance and accurate readings.
The Pt100 RTD sensor can measure temperatures ranging from -330°F (-201°C) to 1560°F (848°C). This broad temperature range makes it versatile and suitable for a wide array of industrial applications.
Temperature sensors are devices that detect and measure coolness and hotness and convert it into an electrical signal. Temperature sensors are utilized in our daily lives, be it in the form of domestic water heaters...
A thermistor, a shortened version of the term thermal resistor, is a passive component whose resistance changes as the temperature in a system changes. Thus they serve as an inexpensive, accurate, and dynamic method for measuring temperature...
A thermocouple is a transducer that converts thermal energy into electrical energy and is constructed by joining wires made from dissimilar metals to form a junction. Voltage is produced when the temperature at the junction changes...
A thermowell is a pressure-tight vessel that safeguards and increases the lifespan of temperature sensors in processing plants in cases where a measuring sensor is not otherwise mechanically or chemically useful in...
A band heater is a heating device that clamps onto objects to provide external heat using radiant and conductive heating. The different mounting methods of band heaters makes it possible to secure them tightly and...
A cartridge heater is a cylindrical tubular heating device that provides concise and precise heating for various forms of materials, machinery, and equipment. Unlike an immersion heater, a cartridge heater is inserted into a hole in the item to be heated to furnish internal radiant heat...
Ceramic heaters are electric heaters that utilize a positive temperature coefficient (PTC) ceramic heating element and generate heat through the principle of resistive heating. Ceramic materials possess sufficient electrical resistance and...
Electric heating is produced by using a known resistance in an electric circuit. This placed resistance has very few free electrons in it so it does not conduct electric current easily through it. When there is resistance in...
A flexible heater is a heater made of material that can bend, stretch, and conform to a surface that requires heating. The various forms of flexible heaters include polyimide film, silicone rubber, tape...
A heating element is a material or device that directly converts electrical energy into heat or thermal energy through a principle known as Joule heating. Joule heating is the phenomenon where a conductor generates heat due to the flow of electric current...
An immersion heater is a fast, economical, and efficient method for heating liquids in tanks, vats, or equipment. Known as bayonet heaters, they have heating elements that can be directly inserted into a container of water, oil, or other material in order to heat the entire contents...
Infrared heating is a heating method used to warm surrounding bodies by infrared radiation. Thermal energy is transferred directly to a body with a lower temperature through electromagnetic waves in the infrared region...
Radiant heaters are systems that generate heat internally and then radiate it to the nearby objects and people. The sun is a basic example of a radiant heater. When we feel warm on our bodies on a sunny day...
The idea of an electric heater seems to be out of place in modern society since most buildings have a sophisticated central heating system. That may be true, but electric heaters can be a helpful way of saving energy while providing efficient heating...