RTD Sensors
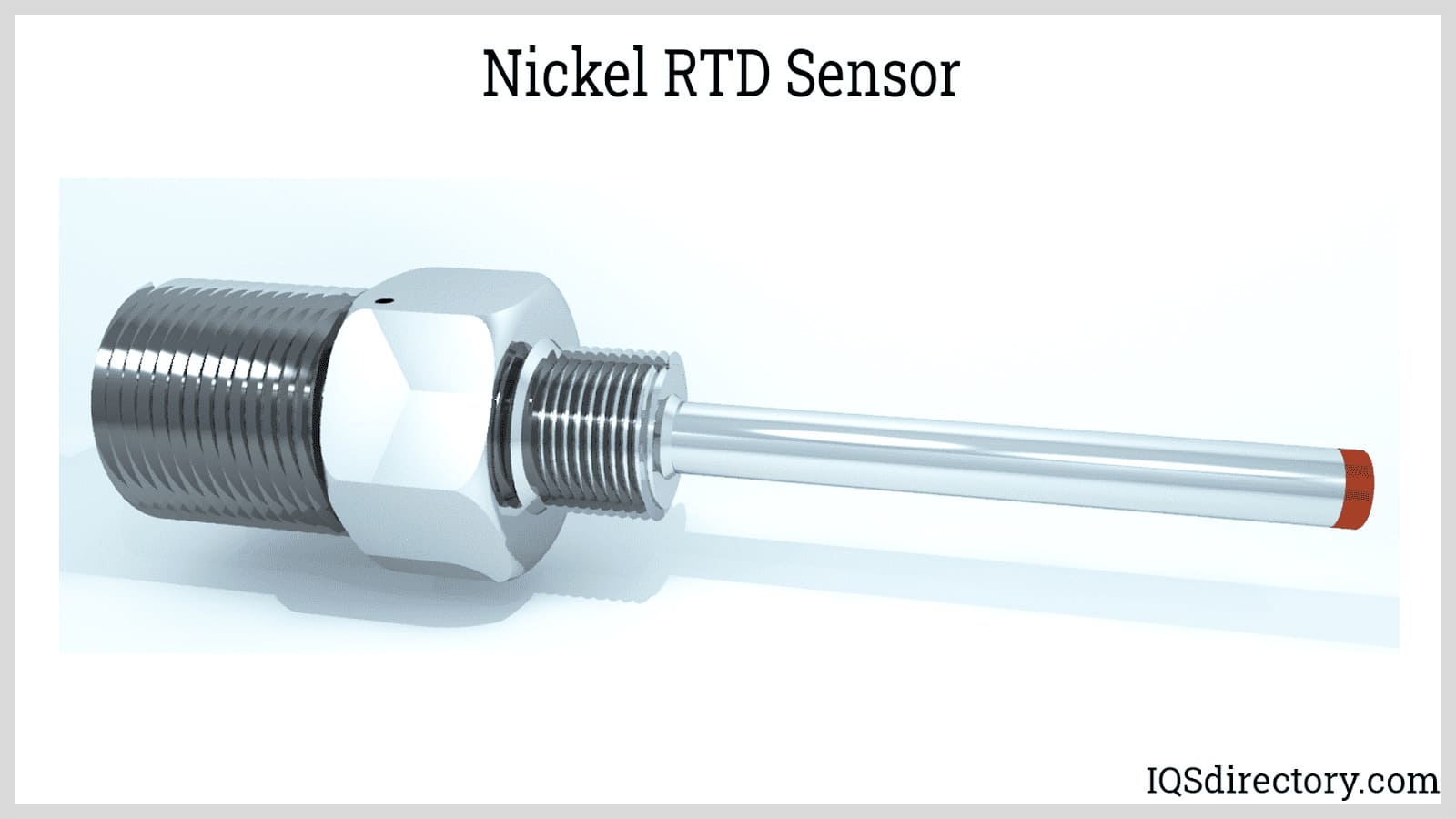
An RTD, resistance temperature detector, is a passive temperature sensing device that operates on the principle that the resistance of a metal changes as the temperature changes. The electrical current that passes through the element...
Please fill out the following form to submit a Request for Quote to any of the following companies listed on
This article will give more detailed information regarding thermowells on topics such as:
This chapter will provide an overview of thermowells, including their definition and function. It will delve into how thermowells operate and outline their critical role in various industries. By understanding these aspects, readers will gain insight into the significance of thermowells in ensuring accurate temperature measurements and maintaining system integrity.
A thermowell is a pressure-tight vessel designed to protect and extend the lifespan of temperature sensors in processing plants, particularly in environments where direct sensor placement is impractical due to mechanical or chemical constraints. Installed directly into piping systems, thermowells facilitate the replacement of sensors in high-pressure pipelines without disrupting process flow or requiring the draining of the processing system. Additionally, standardized thermowells enable easy removal and maintenance of sensors throughout the plant, streamlining the overall process.
Temperature sensors, which include bimetal thermometers, thermocouples, and thermistors, are shielded from corrosion, high material velocities, and extreme pressure by thermowells. This protection not only extends the lifespan of the sensors but also allows for their replacement without the need to empty the system, thus minimizing the risk of contamination. For high-pressure applications, thermowells are typically machined from bar stock to ensure integrity, while smaller thermowells for low-pressure environments can be fabricated from tubing with one end welded shut.
A thermowell separates the sensing element of a temperature measurement device and a process media (a material being processed). It guards against corrosive process media and substances that are pressure-contained or moving quickly. A thermowell also makes it possible to remove the sensing component from a particular application while keeping the system closed. A thermowell may be employed in an application as simple as a cooking pot used in the food processing industry or as complex as a boiler in a brewing industry where temperature is a critical measurement.
Thermowells play a crucial role in industrial applications that demand precise temperature measurement. They provide essential protection for temperature sensors against harsh process conditions, such as corrosion, high velocities, and extreme pressures, ensuring reliable and accurate readings.
To choose the most suitable thermowell for any application, several specifications need to be considered. Important factors to evaluate include material compatibility, pressure and temperature ratings, length and diameter, insertion depth, and end connection type. Additionally, it is crucial to ensure that the thermowell complies with relevant design standards and is durable enough for the application’s specific conditions.
Thermowell Root Dimension (Q): This refers to the thickest part of the thermowell that is inserted into the pipe wall. It is crucial because it correlates with both the size of the process connection and the diameter of the thermowell bore.
Thermowell Immersion Length (U): The thermowell immersion length is the distance from the tip of the thermowell to the base of the process connection.
Thermowell Bore Size (V): The thermowell bore size refers to the internal diameter of the thermowell. Standard bore diameters are typically 0.260" and 0.385", which are designed to fit sensors with corresponding diameters of 0.25” or 0.38”.
Thermowell Lagging (or Trailing) Extension Length (T): The thermowell lagging extension length is the portion of the thermowell that extends beyond the pipe wall after installation. This length is typically an extension of the thermowell's hex length and is defined by the cold head of the process connection.
The Hex Length (P): The hex length measurement is the distance from the top of the mounting threads to the tip of the stem.
Thermocouple Probe: A thermocouple probe consists of a metallic tube housing thermocouple wire. Due to the lagging extension length, the probe and thermowell can extend through insulation or walls. The tube wall of the probe is referred to as the sheath, which is commonly made from steel or other materials.
When installing a thermowell through insulation on a pipeline or equipment, lagging (heat-insulating material for pipes) is used. To prevent potential failures of thermowells, the following factors should be considered:
It's also crucial that the gap between the thermowell's internal diameter (ID) and the thermocouple sheath's outer diameter (OD) is minimal to ensure accurate temperature measurements and avoid potential inaccuracies. Additionally, the thermowell's bore should be uniform and smooth to facilitate the easy insertion of the thermocouple during installation.
Thermowells must be designed to handle specific process media and varying conditions such as pressures, temperatures, velocities, and specific gravities. Additionally, a well-functioning thermowell design should consider the following factors:
Thermowells come in various types, classified by their end connectors—the parts that interface with a measuring sensor's connector. The choice of thermowell depends on the connector type used by the measuring sensor. Below is a list of end connector types, which will be explained in more detail:
Threaded Thermowells: As the name implies, threaded thermowells are screwed directly into the wall of a tapped pipe. These thermowells are generally the most cost-effective and versatile option. They are commonly used in non-corrosive applications, such as measuring the temperature of molten iron, where protecting the thermowell threads from rust is crucial. Due to their material properties, threaded thermowells can also be brazed or welded to enhance their strength and durability.
Socket-Welded Thermowells: Socket-welded thermowells offer a robust and permanent connection, making them ideal for applications involving extremely high temperatures and pressures. The socket-welded joint provides a secure connection that resists pressure and withstands high temperatures. Additionally, in industries such as food and pharmaceuticals, where thread impurities must be avoided, socket-welded thermowells are preferred. This is because the welded socket joint eliminates the risk of impurities or air entering the process, ensuring the integrity of the food or medicine being produced.
Van Stone Thermowells: Van Stone thermowells are well-suited for high-pressure applications due to their robust construction, which allows them to withstand significant forces. Typically, a Van Stone thermowell is secured between a cover flange and nozzle, and is machined from solid bars. The flange surface features a phonographic or continuous spiral serration, which provides multiple grades of surface polish. This design not only enhances the thermowell's strength and durability but also ensures a precise and reliable fit in high-pressure environments.
Scruton Thermowells: To mitigate damage from mechanical loads and address process criticality—based on a company’s risk matrix study—a Scruton thermowell is designed using the ScrutonWell® design. This design incorporates a helix on the outside of the thermowell, facilitating quick and easy installation without the need for a support collar. This approach not only prevents costly and time-consuming site rework but also reduces oscillation amplitude by over 90%. Additionally, the ScrutonWell® design allows for various grades of surface polish, saving both time and money on site rework.
Sanitary Thermowell: A sanitary thermowell isolates and protects the sensing element of a temperature sensor. These thermowells are designed with hygienic connections to prevent microbial growth.
Various methods are used to connect thermowells to piping systems, and these connections can help identify the type of thermowell. Here are some common types of thermowell connections:
Flanged Thermowells: A flanged thermowell features an extended "collar" that offers enhanced support by securing the thermowell more firmly to the pipe.
Weld-in thermowells: Weld-in thermowells are directly welded into a pipe or process vessel, providing the highest quality connection available. This permanent installation method ensures robust performance and is ideal for high-temperature and high-pressure applications where access for maintenance is not required and corrosion is not a concern. Weld-in thermowells are commonly used in systems handling non-corrosive media.
Threaded Thermowells: Threaded thermowells are commonly used in smaller pipelines carrying non-corrosive fluids. They are screwed into the pipe and are not intended for frequent replacement. Below are some typical applications where threaded thermowells are frequently employed:
Threaded thermowells are favored in these applications because they ensure a tight fit, preventing air or water from entering and causing corrosion inside pipes or equipment. This also helps avoid contamination in manufacturing processes.
There are four distinct stem design shapes for thermowells, each suited to specific applications:
The diameter of a straight thermowell remains uniform along its entire length, making it straightforward to manufacture. This design provides excellent stiffness and protection against erosion and corrosion. In contrast, stepped thermowells feature a graduated diameter, typically with a larger diameter at the top (often 3/4") and a smaller diameter at the tip (commonly 1/2"). This design facilitates smoother flow and faster temperature response due to reduced thermal inertia at the process end.
A tapered thermowell features a gradually changing diameter along its entire insertion length, providing a smooth, continuous taper. This design enhances response time, making it ideal for heavy-duty applications where quick temperature measurements are needed due to its reduced wall thickness. On the other hand, built-up thermowells are designed for applications requiring long insertion lengths. These thermowells incorporate a pipe section welded between the tip and process connection, allowing for deeper insertion into vessels with larger liquid volumes. This construction is particularly useful for thermocouples that need to measure temperatures in extended or deep vessels.
In addition to classifying thermowells by their end connections, they can also be categorized based on the materials used in their construction and their shank design. The material choice affects factors like durability and resistance to corrosion, while the shank design—such as solid, tapered, or stepped—determines the thermowell's performance in various applications.
Bar Stock Thermowells: These thermowells are crafted from bar stock, a raw, purified metal that is machined and drilled without the need for welding.
Thermowell Classification by Type of Shank: TThe shank of a thermowell is the part that is inserted into a process piping system or vessel. Common types of shanks include straight, stepped, and tapered designs. A straight-shank thermowell maintains a constant diameter along its immersion length. Step-shank thermowells feature varying diameters along the immersion length, which allows for quicker reaction times. Tapered thermowells have a diameter that gradually decreases along their immersion length. Historically, the ASME PTC 19.3 standard (1974) provided outdated definitions for tapered thermowells, leading to the development of the heavy-duty tapered thermowell for more demanding applications. This type is specifically designed for high-pressure and high-temperature environments and is commonly used in heavy-duty settings where thermowells are threaded into or welded to flanges, or welded directly into pipes or process vessels. For applications where resistance to velocity-induced resonance is crucial, and the inner nozzle diameter is a design constraint, the straight shank thermowell is often the most suitable choice.
Lagging extension is another category of shank thermowells. As detailed in Chapter Two, lagging extension refers to the length of a thermowell that remains exposed after it has been inserted into a piping system. These thermowells are specifically designed for use in conjunction with insulation, allowing them to extend through the insulating material that covers the vessel or piping system.
To ensure that a thermowell can handle the hydrostatic pressure limits and dynamic static stress associated with process conditions, several important calculations are conducted. One key calculation is the wake frequency, which is determined by the ratio of the Strouhal frequency to the natural frequency. According to the ASME PTC 19.3 standard, this ratio must not exceed 0.8 Hz. These calculations are performed prior to manufacturing to validate the thermowell's design and ensure it can withstand the stress and strain imposed by the process media. The Strouhal frequency, which describes oscillating flow mechanisms, is calculated using the formula \( \text{St} = \frac{f_{st}D}{U} \), where \(\text{St}\) is the Strouhal number, \(f_{st}\) is the vortex shedding frequency, \(D\) is the diameter of the circular cylinder, and \(U\) is the ambient flow velocity. In the final phase, the thermowell length is assessed using steady-state stress analysis.
As fluid flows past a thermowell, it creates a turbulent wake behind it due to changes in fluid momentum. This wake results in vortices forming and shedding from various sides of the thermowell. The vortex shedding frequency, or wake frequency, is inversely related to the diameter of the thermowell’s tip and directly proportional to the flow velocity. These shedding vortices generate periodic forces on the thermowell, comprising:1. **Lift Force**: Parallel to the flow direction, this force oscillates at twice the wake frequency.2. **Drag Force**: Perpendicular to the flow direction, this force is less significant compared to the lift force.Typically, these vortex-induced forces are modest, causing only minor vibrations. However, if the wake frequency (FW) approaches the thermowell's natural frequency (fn) within 20%, resonance can occur. When the wake frequency equals the natural frequency (FW = fn), vibrations can increase dramatically, potentially leading to mechanical failure of the thermowell. The ASME PTC 19.3 standard addresses thermowell vibration by primarily considering the oscillating lift force, setting a maximum wake-to-natural frequency ratio of 0.8 Hz to avoid resonance. Although the oscillating drag force is usually minimal, it occurs at twice the wake frequency and can induce resonance at lower speeds. The Murdock analysis, which often underestimates the impact of the drag force, may lead to a reduction in the velocity rating by up to 50% in high-density fluids like liquids and high-pressure steam. Therefore, the results from these calculations should be used as a guideline for selecting the appropriate thermowell rather than the sole determinant.
As fluids flow past a thermowell, they create swirling patterns and low-pressure eddies downstream of the well. These mini whirlpools then detach from various sides of the well, generating two periodic forces on the thermowell.
Vortex shedding can occur within a frequency range of 50 Hz to 1500 Hz. The force generated by vortex shedding increases with the square of the fluid velocity, while the shedding frequency, known as the Strouhal frequency, rises linearly with fluid velocity. If this Strouhal frequency approaches the natural frequency of the thermowell, resonance can occur, leading to significantly amplified forces. To prevent lock-in, the thermowell's natural frequency must be higher than both the in-line and transverse resonance conditions. Operation at in-line resonance is only permissible if the cyclic stresses remain within acceptable limits.It's important to monitor the fluid velocity at which resonance occurs. For each natural frequency of the thermowell, two critical velocities must be considered: one for transverse response and one for in-line response. The critical velocity for transverse resonance is approximately twice as high as that for in-line resonance because the in-line forces fluctuate at twice the frequency of the transverse forces. Significant increases in vibration amplitude can occur if the thermowell's natural frequency aligns with the Strouhal frequency or its harmonic (2fs). Resonance-induced fatigue is a major cause of thermowell failure. However, with adequate damping, a thermowell can operate safely even at in-line or transverse resonance frequencies.
The lifespan of a thermowell is heavily influenced by the material used in its construction. Key factors to consider when selecting a material include the operating temperature, the types of chemicals the thermowell will be exposed to, and the flow rate it will encounter. Chemicals can accelerate corrosion, particularly at elevated temperatures and concentrations, while suspended particles in the fluid can cause erosion. Here is a list of some of the most commonly used materials for thermowell construction:/p>
Carbon steels can only be used in low-temperature, low-pressure applications because of their low resistance to corrosive chemicals. The most-common material used to create thermowells is stainless steel. Thermowells made from stainless steel are affordable and have high heat and corrosion resistance. One high-strength steel used for pressurized vessels is chromium/molybdenum steel. Molybdenum increases corrosion resistance when added to chromium. The elements cobalt, nickel, chromium, and tungsten make up the Haynes alloy®. It is most frequently utilized during carburizing heat treatment, and in chlorine or sulfide conditions.
Insertion Length: The distance from the thermowell's connection point to the tip is known as the insertion length. The insertion length should be long enough to allow the entire temperature-sensitive section of the measuring device to extend into the measured medium in order to attain the highest level of accuracy. A minimum of one inch should be added to the length of the temperature-sensitive part when using a temperature sensor to measure the temperature of liquids. When the length of the temperature-sensitive segment will be submerged in gas or air, an additional three inches should be added. A thermocouple or thermistor can use a thermowell with a lower insertion length since its temperature-sensitive region is shorter. For acceptable accuracy, the temperature-sensitive portion of bimetal thermometers, RTDs, and liquid-in-glass thermometers must be submerged at least 2½ " in liquids.
In which industrial fields are thermowells used, and what benefits do they provide? Thermowells are employed in industries such as petrochemical, food processing, refining, cosmetics, chemicals, power generation, and pharmaceuticals. They protect equipment from external forces and disturbances, including pressure, abrasion, vibration, and corrosion from the processed medium. Additionally, thermowells allow for the removal and replacement of sensors without causing disruptions to the manufacturing environment.
How do thermowells enhance temperature measurement processes and contribute to cost savings? Thermowells offer significant benefits by protecting against corrosion, high material velocities, and extreme pressure damage. They also facilitate the replacement of temperature sensors without needing to drain the system. In summary, thermowells are designed to provide cost savings in various industries. Below is a summary of factors to consider regarding a thermowell’s cost-saving potential.
Longevity: How are thermocouples and RTDs typically protected, and what role do thermowells play in extending their lifespan? Thermocouples and RTDs are often housed in 1/4" diameter stainless steel probes, which provide adequate protection in relatively inert environments. However, in more hostile conditions, such as those involving temperatures above 1000°C (1832°F), the stainless steel can become stressed and the sensor probe may warp. The process medium itself can also be harsh, with corrosive substances like acids or seawater, or solid particles in gases, further challenging the probe's durability. High pressures can exacerbate the negative effects of high temperatures and corrosive environments. Thermowells significantly extend the lifespan of temperature sensors by absorbing and mitigating these harsh conditions.
Sensor Replacement Cost Analysis: How does the cost of temperature sensors and the protection offered by thermowells impact their overall value? The cost of RTDs, thermocouples, or thermistors can vary widely based on the sensor type and its susceptibility to degradation under harsh conditions. The protection provided by a thermowell becomes particularly valuable if the sensors are expensive or operate in demanding environments. Although thermowells represent an additional expense, this cost is often outweighed by the savings achieved through reduced sensor replacement frequency. Conducting a cost-benefit analysis can help determine when investing in a thermowell shifts from being a discretionary expense to a necessary investment, similar to other manufacturing decisions.
Labor Costs: Even if a temperature sensor is inexpensive, adding a thermowell can still be practical. Frequent replacement of sensors incurs labor costs, as sensors will eventually need to be replaced. Replacing sensors is more straightforward when they are housed in a thermowell. Furthermore, a thermowell seals the process, preventing outside contamination during sensor replacement, and eliminating the need for additional protective equipment or measures for workers.
No Shut-Downs: One significant benefit of thermowells is their ability to keep the temperature-monitoring process independent from the temperature sensor itself. Since temperature sensors eventually need to be removed and replaced, the thermowell ensures that the process remains contained during this maintenance. As a result, other than a temporary lapse in temperature measurement, the operation is unaffected by the sensor swap. Without a thermowell, there is a risk of partial or complete disruption of the process during sensor replacement. When deciding whether to use a thermowell, it's important to consider the cost or impact of such disruptions. Additionally, thermowells can be coated with FEP (fluorinated ethylene propylene) for added protection.
Benefits of FEP (Fluorinated Ethylene Propylene) Coated Thermowells: A thermowell can be constructed from various materials. However, to ensure optimal protection, the following factors should be considered during the decision-making process:
FEP (fluorinated ethylene propylene) offers several beneficial properties, making it an ideal material for encasing a thermowell. The advantages of FEP include:
FDA-approved FEP-coated thermowells are versatile and available in lengths of any size with diameters ranging from 1mm to 50mm. For applications that require faster temperature response or involve complex shapes, spray coatings such as PVDF (polyvinylidene fluoride) and PTFE (polytetrafluoroethylene) are effective. These coatings are particularly suitable for instruments exposed to environmental conditions, as they have a low thermal expansion coefficient (0.000122 mm per degree Celsius) and absorb minimal moisture (less than 0.01%). Additionally, coated thermowells are resistant to ultraviolet radiation, providing added protection to the sensor element inside.
Thermowells are designed to meet recognized standards based on established process conditions, but processes are often subject to unexpected changes. For instance, when working with gases or oils, initial assumptions about the source material might evolve due to various external factors. For example, if sand inadvertently enters a piping system, it can start to degrade the thermowell from within the pipe. Additionally, the sensor housed within the thermowell may not always detect or measure the specific materials flowing through, potentially leading to severe failures. Despite careful planning and engineering, thermowells might not always adapt to all process changes. Another limitation is that some thermowells are only compatible with specific types of sensors.
All temperature-monitoring equipment is prone to degradation from flow, heat, and pressure exposure. A severe processing environment will impact a sensor's structural integrity and performance over time. For instance, the metals used to construct thermocouple probes are susceptible to the corrosive conditions where they are typically employed. Under such harmful conditions sensors often begin to deteriorate quickly and, also, may report false readings while they begin to fail. Thermowells protect a sensor’s lifespan and, by doing so, lower overall production costs for the industries they are employed by eliminating the need for regular sensor replacements and the associated material (sensor replacement), labor, and operational costs.
An RTD, resistance temperature detector, is a passive temperature sensing device that operates on the principle that the resistance of a metal changes as the temperature changes. The electrical current that passes through the element...
Temperature sensors are devices that detect and measure coolness and hotness and convert it into an electrical signal. Temperature sensors are utilized in our daily lives, be it in the form of domestic water heaters...
A thermistor, a shortened version of the term thermal resistor, is a passive component whose resistance changes as the temperature in a system changes. Thus they serve as an inexpensive, accurate, and dynamic method for measuring temperature...
A thermocouple is a transducer that converts thermal energy into electrical energy and is constructed by joining wires made from dissimilar metals to form a junction. Voltage is produced when the temperature at the junction changes...
A band heater is a heating device that clamps onto objects to provide external heat using radiant and conductive heating. The different mounting methods of band heaters makes it possible to secure them tightly and...
A cartridge heater is a cylindrical tubular heating device that provides concise and precise heating for various forms of materials, machinery, and equipment. Unlike an immersion heater, a cartridge heater is inserted into a hole in the item to be heated to furnish internal radiant heat...
Ceramic heaters are electric heaters that utilize a positive temperature coefficient (PTC) ceramic heating element and generate heat through the principle of resistive heating. Ceramic materials possess sufficient electrical resistance and...
Electric heating is produced by using a known resistance in an electric circuit. This placed resistance has very few free electrons in it so it does not conduct electric current easily through it. When there is resistance in...
A flexible heater is a heater made of material that can bend, stretch, and conform to a surface that requires heating. The various forms of flexible heaters include polyimide film, silicone rubber, tape...
A heating element is a material or device that directly converts electrical energy into heat or thermal energy through a principle known as Joule heating. Joule heating is the phenomenon where a conductor generates heat due to the flow of electric current...
An immersion heater is a fast, economical, and efficient method for heating liquids in tanks, vats, or equipment. Known as bayonet heaters, they have heating elements that can be directly inserted into a container of water, oil, or other material in order to heat the entire contents...
Infrared heating is a heating method used to warm surrounding bodies by infrared radiation. Thermal energy is transferred directly to a body with a lower temperature through electromagnetic waves in the infrared region...
Radiant heaters are systems that generate heat internally and then radiate it to the nearby objects and people. The sun is a basic example of a radiant heater. When we feel warm on our bodies on a sunny day...
The idea of an electric heater seems to be out of place in modern society since most buildings have a sophisticated central heating system. That may be true, but electric heaters can be a helpful way of saving energy while providing efficient heating...